
News
A paper on cement sheath performance under thermal cycles was published in Energies, 2024, 17, 239
Posted January 31, 2024
https://doi.org/10.3390/en17010239 "The Sealing Performance of Cement Sheaths under Thermal Cycles for Low-Enthalpy Geothermal Wells" By Anisa Noor Corina and Al Moghadam
A paper on cement testing under cyclic temperatures and drying shrinkage was published in Materials 2023, 16, 7281
Posted January 31, 2024
https://doi.org/10.3390/ma16237281. "Testing Various Cement Formulations under Temperature Cycles and Drying Shrinkage for Low-Temperature GeothermalWells" By Hartmut R. Fischer and Al Moghadam
The work on supercritical cement will be presented at Stanford geothermal workshop, February 12-14, 2024.
Posted January 31, 2024
"Aluminum-rich cements for high temperature geothermal wells" By T. Pyatina and T. Sugama
A preprint of the paper “Assessment of cementitious composites for high temperature geothermal wells” by Tatiana Pyatina, Toshifumi Sugama, Al Moghadam, Marcel Naumann, Ragnhild Scorpa, Blandine Feneuil, Vincent Saustelle, Rune Godøy was published
Posted January 31, 2024
The modeling work was presented at the SPE Offshore Europe Conference & Exhibition, Aberdeen, Scotland, UK, September 2023
Posted Sept. 15, 2023
The progress of the project was presented in two papers at GRC, October 1-4, 2023, Reno, Nevada, US
Posted April 12, 2023
The results of pressure functional tests of two supercritical cement formulations will be presented at the 42nd International Conference on Ocean, Offshore & Arctic Engineering, June 11-16, Melbourne Australia
Posted April 12, 2023
The modeling work on low-to-medium temperature range cements will be presented at SPE EuropEC – Europe Energy Conference, 5-8 June 2023, Vienna, Austria
Posted April 12, 2023
The TEST CEM project presented at 56th US Rock Mechanics / Geomechanics Symposium
Posted August 15, 2022
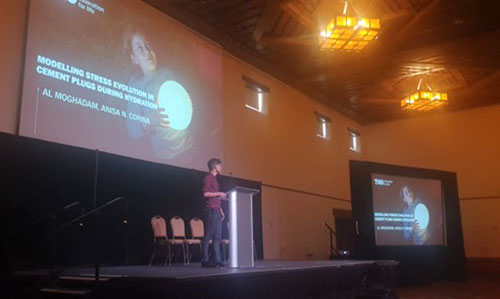
The TEST CEM project was presented by Al Moghadam (TNO) at the 56th US Rock Mechanics / Geomechanics Symposium (Santa Fe, 26th-29th June 2022).
A methodology to model the stress evolution in cement plugs during hydration was presented.
New knowledge on cement behavior under lab and field conditions was shared and discussed, as for example how understanding the mechanisms responsible for the cement stress drop helps in designing better cement recipes that can maintain higher initial stress levels.
The well integrity modeling work was presented at the Celle Drilling 2022 conference (September 13-14)
Posted July 6, 2022
Al Moghadam, TNO
Assessing the Impact of Temperature Cycles on well Cement in Low Enthalpy Geothermal Wells.
Current work on supercritical cement was presented at the Stanford Geothermal Workshop
Posted Nov. 1, 2021
This work is a part of a joint international effort to develop “sustainable geothermal well cement for challenging thermo-mechanical conditions” (Test-Cem) under the umbrella of Cofund GEOTHERMICA. The paper (below) describes an exploratory study of cementitious composites for applications in geothermal wells under super-critical conditions.