Magnetic Measurements Question Assumptions About High-Tc Superconductors
Quest for understanding high-Tc materials that carry current with no resistance continues
August 2, 2009
UPTON, NY — Conquering one of the biggest challenges in the study of high-temperature (high-Tc) superconductors, scientists at the U.S. Department of Energy’s (DOE) Brookhaven National Laboratory have grown crystals of one such material that are large enough to directly measure the material’s magnetic properties. These measurements, published online on August 2 by Nature Physics, cast considerable doubt on some assumptions commonly made in trying to understand the role magnetism plays in these materials’ ability to carry current with no resistance. Such materials promise more-efficient, lower-cost energy transmission if they can be made to operate under real-world conditions.
“Many theorists believe that magnetism is important for high-temperature superconductivity, although they don’t agree on how it is important,” said Brookhaven physicist John Tranquada, who led the research team. Figuring out this puzzle has been complicated by the fact that techniques used to measure materials’ magnetic properties require good-quality, large, single crystals — and growing such crystals of high-Tc materials has been a long-term challenge.
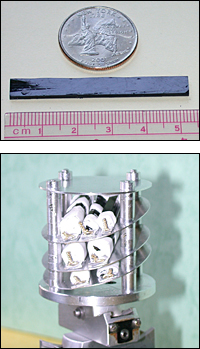
Top: A single crystal of BSCCO showing the typical size achieved by the Brookhaven team. Bottom: To study the magnetic properties of BSCCO, the scientists aligned several crystals in a specialized holder that was then placed in the path of a neutron beam. Measurements of beam scattering revealed details about the magnetic fluctuations in the material over a range of temperatures.
Smaller crystals work well for studies of electronic properties, however, so those properties have been characterized for select high-Tc superconductors. Since magnetic properties in conventional metallic conductors are a direct result of those materials’ electronic properties, theorists have used the same well-established mathematical approach for deriving magnetism from electronic measurements in high-Tc materials. The Brookhaven team’s success at finally growing large crystals of a well-studied high-Tc material offered the first opportunity to directly test the assumption that this approach is valid.
“The calculations based on the material’s electronic properties — which change dramatically as the material is cooled and transitions from its electrically resistive state to become a superconductor — predicted there would be a similar large change in magnetic characteristics below the transition temperature (Tc),” said Brookhaven physicist Guangyong Xu. “But our direct measurements of the magnetic properties showed surprisingly little change. This implies that the model the theorists have been using to describe these magnetic properties is incomplete.”
It’s not that the magnetic properties are completely unrelated to the electronic properties; they are both still part of the same system, the scientists emphasize. Magnetism, after all, comes from the relative arrangements of the directions in which electrons spin, like a collection of tiny bar magnets.
“It could be that the magnetism somehow drives the electronic structure, rather than the other way around — or that something underlying both magnetism and electronic structure influences both but in different ways,” Xu said.
“You can think of it as the foreground and the background of a painting,” Tranquada suggested. “We are interested in the superconductivity, which is what stands out — the foreground. And we know electrons are involved in that by pairing up to carry current with no resistance. But are those same electrons defining the magnetic properties? Or do other, ‘background’ electrons define the magnetism?”
The magnetic measurements showed that some of the magnetic characteristics of the original “parent” compound — which is an insulator — remain when the material becomes a superconductor. This suggests that there may be two kinds of electrons: some moving around like waves to carry the current while others remain in relatively fixed positions to produce the magnetism.
Defining these characteristics will be important as scientists search for or try to design new materials that act as superconductors at temperatures appropriate for real-world applications, such as high-efficiency power transmission lines.
“If the dual existence of localized and free-flowing electrons is important, we want to look for other materials that have those characteristics, but transition to superconductivity at even higher temperatures,” Tranquada said.
This research was funded by the Office of Basic Energy Sciences within DOE’s Office of Science.
Making and Analyzing the Crystals
The Brookhaven team studied the copper-oxide superconductor that has undergone the most extensive electronic analysis of any high-Tc material. Abbreviated as BSCCO, the material contains bismuth, strontium, and calcium in addition to copper and oxygen.
“It’s very easy to produce the small crystals required for electronic studies, but it is very difficult to grow the large BSCCO single crystals with millimeter thickness that are required for magnetic analysis,” said Brookhaven physicist Genda Gu, an expert in crystal growth. “We developed a special technique and operated two specialized furnaces continuously — 24 hours a day/7 days a week — for two years to grow the large crystals used in this study.” “No one else in the world has matched this feat,” said team leader John Tranquada.
To measure the fluctuating magnetism in these crystals, the scientists used a neutron scattering spectrometer at the Rutherford Appleton Laboratory in the U.K. As beams of neutrons are scattered off the crystal sample, detectors pick up subtle changes in the deflected particles’ energy and momentum to reveal information about the sample’s magnetic properties. Vibrations of the crystal lattice can obscure the magnetic fluctuations, so careful analysis is needed to separate the “signal” from this background. The scientists performed additional measurements at the Institut Laue-Langevin in France to verify the identity of the magnetic fluctuations.
Finally, to check the magnetic measurements against predictions made by calculations using data from electronic studies, the scientists looked for changes in the magnetic response over a range of temperature, from the non-superconducting state to below the transition temperature (Tc) where the material becomes a superconductor.
Unlike the dramatic changes observed in electronic behavior as the material is cooled below the transition temperature, there were only minor changes in magnetic behavior. This finding challenges the validity of the most popular theoretical models currently used to predict magnetic properties from electronic measurements.
2009-10989 | INT/EXT | Newsroom