Of Leaves and Light
Models built from plants' optical properties could enable real-time remote monitoring for managing ecosystems and agriculture
August 23, 2019
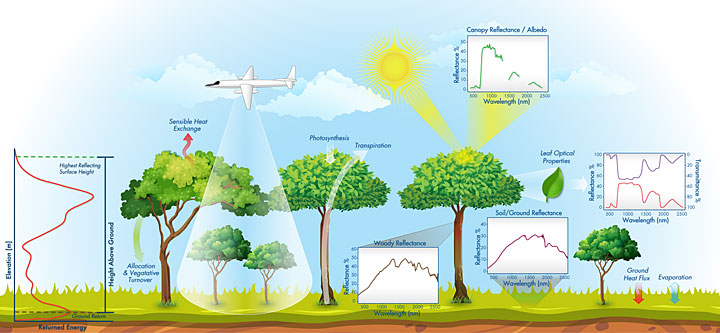
High resolution spectral imaging instruments can collect data on leaf and canopy optical properties that are directly related to leaf biochemistry and morphology, as well as overall canopy structure. When combined with other measurements such as temperature and LIDAR (which measures forest height) these data can map both minor and major variations in the properties of vegetation to provide a full picture of ecosystem structure and health across a landscape.
Imagine getting an entire health workup just by having your picture taken—no invasive poking or prodding, not even a pinprick blood test. That’s a goal ecologists have for monitoring the health of plants. Their cameras would be high-resolution sensors mounted on drones or satellites, capable of capturing much more than what’s visible to the naked eye.
“When you think about what we can see when we look at a leaf, we can see its shape and its greenness, but there is a vast amount of information we can’t see with our eyes that you can see with these instruments,” said Alistair Rogers, leader of the Terrestrial Ecosystem Science & Technology (TEST) group at the U.S. Department of Energy’s Brookhaven National Laboratory.
By tracking wavelengths of light beyond the visible spectrum, tools like spectrometers pick up unique signatures of plants’ biochemical makeup—levels of carbon, nitrogen, proteins, water, and enzymes essential for photosynthesis—all determined by the physics of how light (visible and invisible infrared or ultraviolet light) interacts with these molecules. In three new papers, members of Brookhaven’s TEST group describe how they’ve developed robust models to translate these “spectral fingerprints” into information scientists can use to address challenges in agriculture, ecology, and more.
“If you know exactly what chemicals are in abundance or what plants don’t have enough of, you can target treatments to very specific areas,” said TEST ecologist Shawn Serbin, lead author on one of the papers. “You can target individual plants that are stressed. You can say I know exactly what’s wrong with that plant; it has these chemical deficiencies. Or this insect might be causing some specific changes. You could see a plant being stressed while it still looks green, before any real destruction—in time to make management decisions, in time to make a difference.”
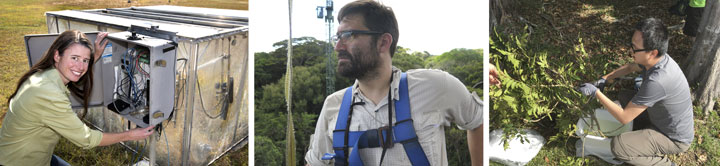
Papers by these three Brookhaven Lab environmental scientists demonstrate the idea of developing models based on spectral measurements that can be used to remotely monitor plant health from the level of individual leaves to entire ecosystems. Left to right: Kim Ely, Shawn Serbin, and Jin Wu.
The models and remote measurements could also help monitor plants’ potential to respond to a changing climate, track biodiversity in forests, and guide agricultural breeding strategies to maximize the productivity of farmland.
“The applications are limitless, and the scale is broad. The key is to be certain we can match information collected remotely from individual leaves or entire ecosystems to what’s actually happening in the plants on the ground,” Rogers said. “These papers establish that we can.”
Building the models
For each of the three papers, the scientists started with data gathered from actual plants—spectral measurements and leaf samples sent to the lab to be ground up for biochemical analyses. They used about two thirds of this information—spectral signals and the corresponding biochemical details and other leaf traits—to build computational models. The models found the statistical connections between the signals and key traits. They then used the resulting models to predict the biochemistry of the final third of samples just by analyzing the plants’ spectral fingerprints.
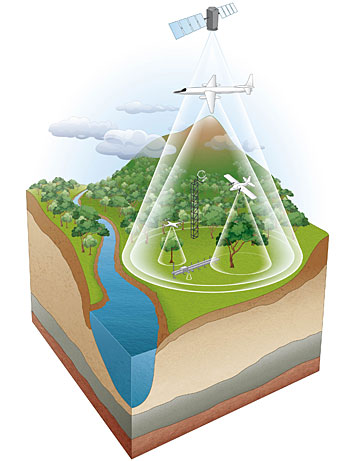
Moving from leaf- to canopy- to landscape-scale, spectral models have to account for increasing complexity arising from the mix of vegetation and its three-dimensional structure across the landscape. The Brookhaven team is addressing this challenge by co-locating and integrating measurements with pixel sizes ranging from centimeters to kilometers that have been collected from instruments mounted on trams, drones, towers, aircraft, and satellites.
The predictions had a high degree of accuracy, giving the team confidence that spectral measurements can be used to give detailed biochemical information—a quick “health check”—without ever needing to collect leaf samples.
“Sampling leaves is great,” Rogers noted. “But if you want to measure a plant throughout its life cycle, you can’t take leaves off every week or you won’t have any plant left. These studies show you can build relationships between the spectral signatures and the leaves’ biochemical properties so you can measure those properties quickly and non-destructively just by scanning the plant with a spectrometer.”
Serbin added, “With spectra, you can do in minutes what would take hours on the ground.”
Plus, with spectrometers mounted on unmanned vehicles or satellites, you could sample large areas again and again to track changes over time.
“We are leading the development of these detailed models because we have the combined expertise. We know how to use the instruments and interpret the signals—and we understand the physiology of plants,” Rogers said.
The three studies
Each of the group’s three papers describes a study that employs this same basic strategy but has a different primary focus.
Brookhaven environmental scientist Kim Ely led a study focused on measuring biochemical compounds that can be used to diagnose the balance of plant nutrients. Scanning the spectral fingerprints of many different varieties would be an efficient way to identify plants with desirable biochemical traits and use that information to guide plant-breeding strategies.
“Traditional methods of analyzing physiological traits are slow, labor intensive, and often destructive,” said Ely. “Using remote sensing for high-throughput screening is especially powerful since it is non-destructive. It enables measurements to be repeated on the same plants throughout a growing season, providing valuable insight into the response of key plant traits to environmental and developmental change.”
Understanding the links between plant traits and growth can guide breeding programs to select appropriate traits for increasing yield. This work could help meet the increasing global demand for food and biofuels, and also help identify plants that are more resilient to climate change.
A second study led by TEST member Jin Wu sought to gain a better understanding of the variation in plants’ ability to take up carbon dioxide (CO2)—their “carboxylation capacity”—through the process of photosynthesis. Representing this variation is essential for predicting how plants of various types, particularly the enormous variety in the tropics, will respond to changing levels of CO2 in Earth’s atmosphere.
The study built upon work funded by the Department of Energy’s Next Generation Ecosystem Experiments-Tropics, or NGEE-Tropics.
“We collected data on leaf gas exchange, reflectance spectroscopy, and age from three lowland seasonal moist tropical forests in Panama and Brazil,” Wu said. “Our goal was to develop a single model capable of capturing the variation in CO2 uptake among leaves of different ages from a range of species and growth environments in the tropics.”
One realization was that the model had to account for leaf age in order for the spectral signals to accurately predict the level of the key enzyme that limits plants’ capacity for CO2 uptake.
“We’ll be able to apply what we learned in this study to develop novel remote sensing approaches for measuring leaf carboxylation capacity throughout the tropics,” Wu said. Such a system will ultimately improve how Earth system models represent plants’ role in the global carbon cycle.
The third study, authored by Serbin, sought to identify a spectral signature for measuring “leaf mass area”—an indicator of how plants balance their resources between building structure (mass) and maximizing the surface area available for capturing sunlight for photosynthesis.
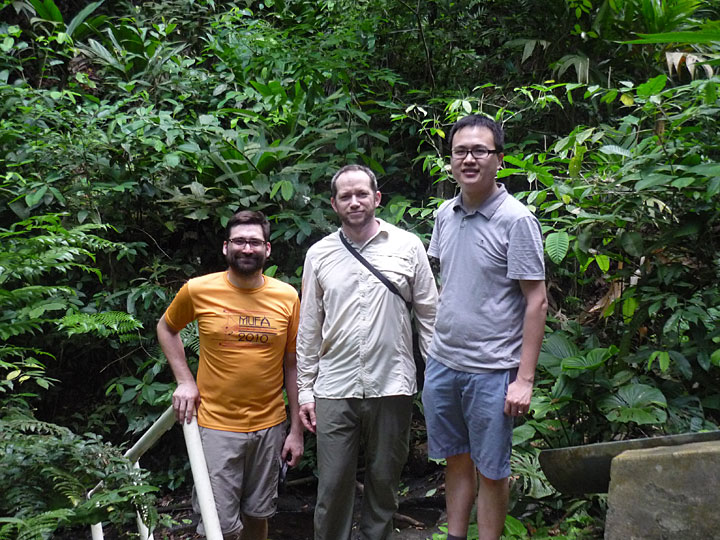
Shawn Serbin, TEST Group leader Alistair Rogers, and Jin Wu take a break from data collection in the field.
Leaf mass area is a key variable used to monitor biodiversity, so Serbin wanted his model to be applicable across a wide range of ecosystems. Such a global model would be extremely valuable for scientists monitoring how plants acclimate to climatic variability and global change. But to build such a universally applicable model, you need a lot of data.
“There is lots of variability even within an individual species, so the model has to have a lot of variability built into it,” Serbin said. “If built well, the model will pick up on those changes because of the connection we have developed between the spectral details of the leaf and its chemistry.”
Serbin’s study relied on plant measurements made by many groups across many different projects, including DOE-funded research in the Arctic and the tropics.
The paper concludes that the predictive value of the leaf mass area model is strong and the scientists have made it available for use by the entire scientific community.
“Anybody could use this model to analyze a spectral measurement and have high confidence that they have an accurate measure of leaf mass area,” Serbin said.
“If you have a satellite-based measurement you can incorporate information over larger ground,” he noted. So the next step will be to work with spectrometers carried by drones and piloted aircraft to bridge those scales—going from leaf-scale, to canopy, to landscape, and ultimately to a grid cell on a satellite image.
Now that the models are in place, the sky—or the ability to launch sampling devices into it—is the limit.
Serbin and Wu’s studies were funded by the U.S. Department of Energy’s Office of Science. Ely’s study was funded by Brookhaven’s Laboratory Directed Research and Development program. Additional details about funding and datasets can be found in each published paper.
Brookhaven National Laboratory is supported by the Office of Science of the U.S. Department of Energy. The Office of Science is the single largest supporter of basic research in the physical sciences in the United States, and is working to address some of the most pressing challenges of our time. For more information, please visit science.energy.gov.
Follow @BrookhavenLab on Twitter or find us on Facebook.
You can also follow the TEST group directly: @TESTgroup_BNL
2019-13279 | INT/EXT | Newsroom