NSLS-II User Profiles: Pankaj Sarin
March 21, 2016
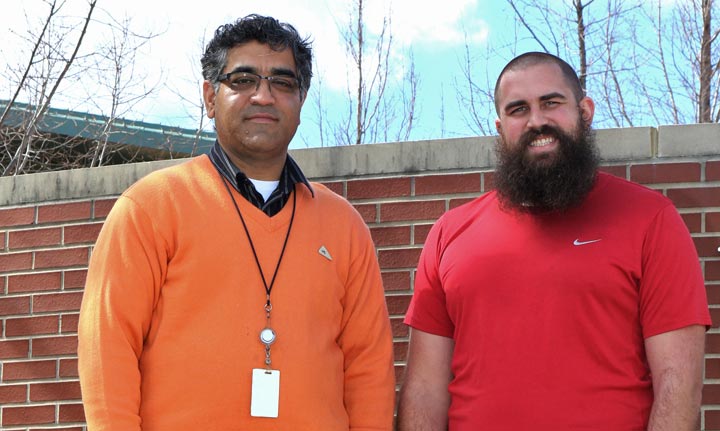
Pankaj Sarin and Dan Lowry are scientific users at the X-ray Powder Diffraction (XPD) beamline at NSLS-II.
Pankaj Sarin, an assistant professor in the School of Materials Science and Engineering at Oklahoma State University, traveled to Brookhaven Lab recently to conduct research at the X-Ray Powder Diffraction (XPD) beamline. He and his group studied ceramic materials that can withstand extremely high temperatures and may be used to protect spacecraft during re-entry, descent, and landing.
Tell us about your research interests.
The goal of my research is to understand the interplay between the atomic structures and intrinsic properties of ceramic materials, as well as these materials' processed microstructures and macroscopic properties. Currently, my research group at OSU is focused on the development of advanced ceramic materials for applications in aerospace, energy generation, energy storage, environmental remediation, and as orthopedic scaffolds.
Ceramics have complex atomic structures and, therefore, offer numerous possibilities for the development of new, exciting functional materials by design. They are suitable for applications in several technologies due to certain key properties, such as stability even at high temperatures; high hardness; a wide range of ionic, electronic and thermal conductivities; and even biocompatibility. To apply these properties to solving some of the most challenging problems worldwide, we need a complete understanding of ceramic material structures, from their atomic structures to their macrostructures, and from static states to the dynamics that occur under the extreme conditions in real-life applications and during processing.
As most ceramic materials are subjected to high temperatures either during processing or incorporated into applications, a substantial part of my research effort is devoted to the development of instrumentation to enable in-situ high temperature studies on ceramic materials using synchrotron radiation. This has allowed me and my group to conduct novel experimental studies to gain a fundamental understanding of the high-temperature phase transformations, thermal expansion, crystallization, melting, microstructure evolution, and oxidation of key ceramic material systems (the last being the subject of our current work at NSLS-II).
What material were you studying at NSLS-II, and why?
The goal of our research at NSLS-II is to develop oxidation-resistant hafnium diboride (HfB2) composites for aerospace applications beyond 2000°C in air. These composites belong to a class of materials usually referred to as ultra-high temperature ceramics, which have high melting points (greater than 3000°C), high hardness, good wear resistance, good mechanical strength, good chemical and thermal stability (under certain conditions), and high thermal conductivity.
A considerable effort in the aerospace industry is focused on the design and development of materials and systems to protect spacecraft from extremely high temperatures during all phases of the mission, including entry, descent, and landing. Reusable thermal protection systems are also key technologies for future hyper-sonic cruise vehicles, which may be used in rapid global and space access missions. For example, it is projected that hypersonic Mach 10 vehicles with sharp aero-surfaces will require materials that can be heated to 2000ºC, can operate in air, and are re-usable. Ultra-high temperature ceramic composites are excellent candidates for these applications.
At NSLS-II, we studied a type of ceramic material that may be resistant to oxidation at high temperatures, which can compromise the material. Specifically, we looked at a sample that could form a dense oxidation-resistant "skin" layer when exposed to oxygen at temperatures around 3000°F, which protects the material from further oxidation. We used the X-ray Powder Diffraction (XPD) beamline to perform x-ray diffraction and total scattering simultaneously, while heating the sample in a water-cooled quadrupole lamp furnace we developed for this purpose.
In-situ x-ray diffraction using synchrotron radiation provides invaluable information toward our understanding of the oxidation of diboride ceramics. A complete understanding of the oxidation mechanism requires the identification of any defect structures as well as molten or amorphous phases and species. For the very first time, it is now possible to identify and quantify any intermediate crystalline and amorphous phases that are formed during the oxidation of HfB2, in real time. The kinetics and energetics of the oxidation reaction will be correlated with the microstructure of the oxidized sample.
Why did you choose NSLS-II for this study?
The oxidation kinetics and evolution of secondary phases in an HfB2 system have never been studied in-situ, and are not well understood. The XPD beamline is particularly suited for this work due to several critical features. These include the high photon flux, particularly for small beam sizes, parallel beam geometry, the available photon energy radiation, the capability to acquire high resolution diffraction and total scattering datasets in fraction of a second using the 2D Flat Panel Detectors, fast switching between diffraction and total scattering modes, and a diffractometer design that allows us to position the quadrupole lamp furnace in the beam path.
Besides the hardware and software capabilities available at XPD, the profound experience of the beamline scientists in extreme-environment diffraction and total scattering experiments has been critical to guiding this research to hugely successful outcomes. In particular, I would like to thank associate physicist Dr. Sanjit Ghose and the XPD group leader, Dr. Eric Dooryhee.
Who collaborated with you on this study?
Daniel Lowry, a graduate student in the School of Materials Science and Engineering at OSU, worked with me on this research experiment. Daniel recently completed his MS degree at OSU and is now working with my group to earn his PhD in materials science engineering. I would also like to acknowledge Dr. Fnu Shikhar Jha, a postdoctoral researcher in the Department of Mechanical Engineering at the University of Colorado at Boulder.
Our research receives sponsorship from the Oklahoma NASA Experimental Program to Stimulate Competitive Research (EPSCoR) program.
2016-6239 | INT/EXT | Newsroom