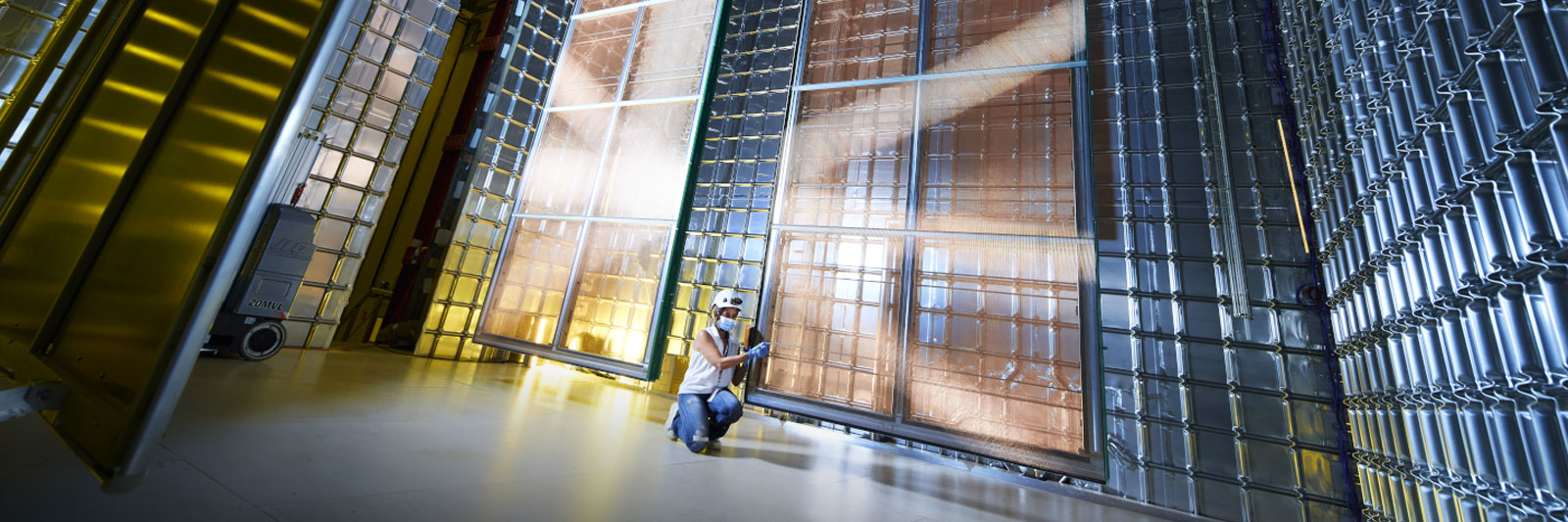
DUNE
The world’s most intense neutrino beam will travel hundreds of miles through Earth’s crust, unraveling mysteries and burrowing back in time
The Deep Underground Neutrino Experiment (DUNE) is a globally organized endeavor to understand the nature of neutrinos—ghostlike particles that travel at nearly the speed of light. With more than 1,000 scientists, DUNE is the largest neutrino experiment collaboration in the world. Brookhaven Lab has played a leading role in DUNE from its inception, contributing to the experiment’s design, construction, operations, and data analysis.
Why Neutrinos?
Neutrinos are fundamental particles most often produced naturally in the fiery hearts of stars, cosmic rays, and supernovae, but also in nuclear reactors and particle accelerators. They are both famously elusive and tremendously abundant. While neutrinos from space endlessly bombard every inch of Earth’s surface at nearly the speed of light, they seldom interact with any matter. This ability to sail unhindered and unnoticed through almost anything earned neutrinos the nickname “ghost” particles. But despite their imperceptibility, neutrinos could be the key to understanding how our universe evolved just after the Big Bang and why the world is made of matter.
One of the most intriguing puzzles in fundamental physics is understanding how neutrinos oscillate between three distinct “flavors”: muon, tau, and electron. The 1988 Nobel Prize in physics was awarded to scientists who discovered the muon neutrino in experiments at Brookhaven Lab’s Alternating Gradient Synchrotron. A second Nobel Prize in physics was awarded to a Brookhaven scientist in 2002 for the detection of solar neutrinos, which revealed the first hint that neutrinos must oscillate between the three known flavors. Once DUNE is operational, scientists will observe neutrinos oscillating as they travel 800 miles underground, across a slice of Earth’s surface and as deep as 40 miles.
Morphing Through Miles of Rock
DUNE will send an intense beam of neutrinos through two particle detectors—the “near” detector at Fermi National Accelerator Laboratory in Batavia, Illinois, and the “far” detector at the Sanford Underground Research Facility in Lead, South Dakota. This 800-mile journey, though not much more than a blink for speedy neutrinos, gives the particles enough time to oscillate. Brookhaven Lab scientists and other DUNE collaborators will measure how the mix of neutrino flavors changes from the start to the end of the beam’s journey. Learn more about the science goals of DUNE.
Brookhaven physicists helped develop the methods for creating neutrinos by striking target materials with a powerful proton beam. They developed simulations to test the beam’s sensitivity and energy range, and also worked with the team designing the trajectory for the resulting neutrinos. Brookhaven scientists were instrumental in developing simulations to determine the sensitivity of the experiment to the pattern of neutrino oscillations.
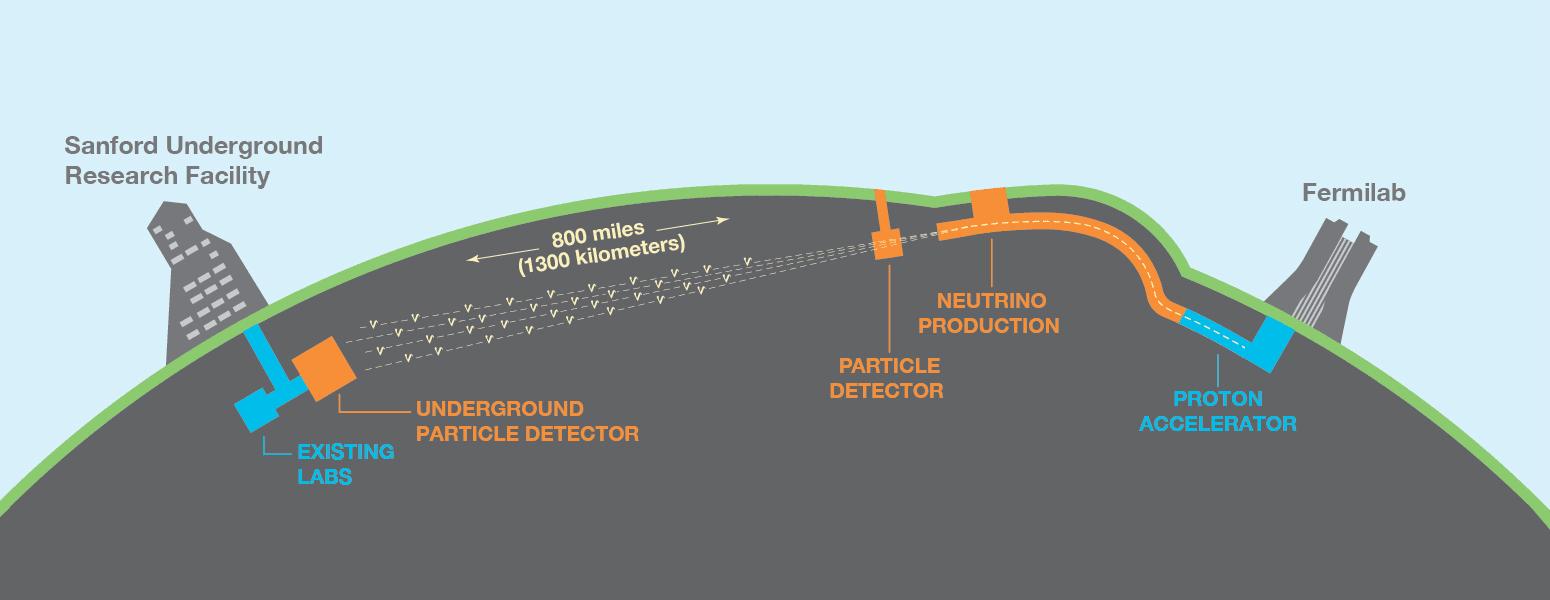
Neutrino production associated with DUNE.
40,000-ton Cryogenic Detector
The neutrino detector at Sanford Lab will be a vast, multi-kiloton, technological marvel filled with frigid liquid argon kept below -300 degrees Fahrenheit—a detector design pioneered by Brookhaven scientists and engineers. As the neutrinos interact with the liquid argon, they create charged particles that ionize individual argon atoms, leaving trails of current like lighning bolts. The type of particle and shape of the trail is determined by the flavor of the passing neutrino. By mapping the tracks, scientists can deduce information about the neutrinos that created them.
Cold Microelectronics
To detect electrical currents in a bath of frigid liquid argon, scientists and engineers at Brookhaven Lab developed specialized “cold microelectronics” that can operate when installed directly inside the ultra-cold liquid. In addition to sensing the signals created by neutrinos’ interactions with argon, these electronics will convert the analog signals into digital ones right inside the detector. Digital signals aren’t susceptible to interference from electromagnetic fields or temperature the way analog electronic signals are. So, once converted from analog to digital, the signals can travel back into the “warm” environment for analysis without interference.
3D Signal Processing
Just like a personal computer requires software to be accessed, physicists need software to access DUNE’s detector and decipher the neutrino data it captures. Brookhaven physicists have contributed years of research to neutrino signal processing software, developing methods to enhance image quality, isolate weak neutrino signals, and differentiate between various neutrino types. The result is a software package called Wire-Cell, which processes raw data from DUNE’s detector and automatically reconstructs neutrino signals in 3D.
Wire-Cell works like the 3D image reconstruction software in a computed tomography (CT) machine. The software package starts by processing 2D snapshots from the detector into high-resolution images. Then, these images are “sliced” into several layers that correspond to a specific moment in time. By combining all the timestamped layers, Wire-Cell creates an interactive 3D model of all the particle data captured by DUNE. Wire-Cell then uses a series of algorithms, including artificial intelligence techniques, to isolate the lightning-bolt-like track of electric charges generated by neutrino interactions.
Computing and Analysis
DUNE's intricate detectors will produce enormous images, generating the equivalent of a 4K high-definition movie in just a few milliseconds. That vast quantity of data must be efficiently calibrated, catalogued, and transmitted to scientists around the world. Physicists will also produce equally detailed simulations of DUNE data, which they will compare to the experimental data to validate their understanding of the detectors and conduct physics analyses. Physicists and computer scientists at Brookhaven Lab are developing the sophisticated computing tools for handling and analyzing these massive datasets.
Brookhaven physicists are among those who will use DUNE's data to study fundamental questions about the universe, such as why the universe is dominated by matter and how the heavier elements that are required for life were produced. To optimize the design of DUNE and to prepare for future analyses that will answer these and other important physics questions, Brookhaven physicists are already performing detailed analyses on simulated data. These studies will determine the expected sensitivity of the experiment so scientists can make detector and experiment design choices—and explore the potential impact of experimental uncertainties on DUNE's results.
The Road to DUNE
Brookhaven scientists have developed and tested Wire-Cell, cold electronics, and other major components of liquid argon time projection chamber (LArTPC) neutrino detectors in a range of neutrino experiments leading up to DUNE.
For example, the MicroBooNE experiment showcased many of the LArTPC technologies, including the cold electronics and Wire-Cell’s ability to reconstruct neutrino tracks. With MicroBooNE, scientists were able to tease out neutrino signals from an array of other particles and pinpoint their vertices, or points of origin—a capability that will be essential to identifying neutrinos at DUNE.
Physicists have also built a smaller version of DUNE’s far detector for testing at CERN, the European laboratory for particle physics, which is another leading collaborator on DUNE. This smaller scale ProtoDUNE detector incorporates the exact components that will be used at Sanford Lab. Though ProtoDUNE does not track neutrinos, scientists have used CERN’s particle beams to put all the particle tracking components to the test—and the detector passed with flying colors. Builiding ProtoDUNE also gave scientists a chance to test all the production and assembly techniques and the quality control procedures that will be used for constructing the full-size DUNE detector.