Big Chill Sets in as RHIC Physics Heats Up
Run 14 promises highest collision rates enabling exploration of detailed properties of early-universe matter
February 3, 2014
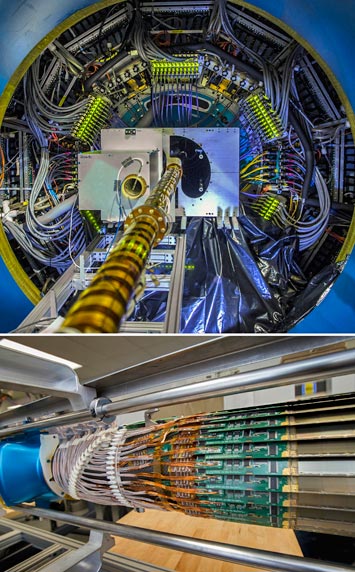
The central portion of the Heavy Flavor Tracker (HFT) being installed at RHIC's STAR detector (top), and the surrounding portion before installation (bottom). The HFT will track particles made of "charm" and "beauty" quarks, rare varieties (or "flavors") that are more massive than the lighter "up" and "down" quarks that make up ordinary matter.
UPTON, NY—If you think it's been cold outside this winter, that's nothing compared to the deep freeze setting in at the Relativistic Heavy Ion Collider (RHIC), the early-universe-recreating "atom smasher" at the U.S. Department of Energy's Brookhaven National Laboratory. Brookhaven's accelerator physicists have begun pumping liquid helium into RHIC's 1,740 superconducting magnets to chill them to near absolute zero (-273 degrees Celsius—the coldest anything can get) in preparation for the collider's next physics run.
Once that extreme subzero temperature is reached, enabling the magnets to operate with zero energy loss, the physicists will begin injecting beams of gold ions and steering them into head-on collisions at nearly the speed of light. Those collisions create temperatures at the opposite extreme of the temperature scale—4 trillion degrees Celsius, or 250,000 times hotter than the center of the sun—to produce RHIC's signature "perfect" liquid quark-gluon plasma, a stand in for what the universe was like an instant after the Big Bang. During this experimental run, the 14th at this nuclear physics scientific user facility, scientists will conduct detailed studies of the primordial plasma's properties and fill in some missing data points to plot its transition to the matter we see in the universe today.
22-week gold-gold run will include 3 weeks at low energy to complete beam energy scan, 15 weeks at high energy for detailed studies of plasma, plus time for cool down and warm up of magnets.
"This run will feature the full complement of accelerator and detector upgrades that constitute the 'RHIC II' program—a dramatic improvement in machine performance that was achieved at a small fraction of the cost and half a decade earlier than originally anticipated," said Berndt Mueller, Brookhaven's Associate Laboratory Director for Nuclear and Particle Physics. "It is particularly fortuitous that we received good budget news that will enable us to take full advantage of these advanced capabilities with 22 weeks of total running time," he said.
According to Wolfram Fischer, Associate Chair for Accelerators of Brookhaven's Collider-Accelerator Department, RHIC will deliver an "integrated luminosity of up to 15 inverse nanobarns"—that's a measure of the number of collisions—during this year's physics run. Compare that with 15 inverse nanobarns produced over the entire set of RHIC heavy ion collisions conducted since operations began in 2000, and you can see why Fischer says, "In terms of physics, this run will be as good as all the previous runs combined."
Accelerator advances
One main reason for the amped up collision rate is a series of innovative techniques RHIC accelerator physicists have installed over past several years to squeeze, "cool," and nudge the ion beams to keep the particles tightly packed. Tightly packed particles collide more often when the beams cross at RHIC's interaction points.
One such technique, called stochastic cooling, uses electronic "pickups" placed around the RHIC ring to measure tiny random fluctuations in the positions of particles as the beams heat up and spread out. These devices send the information across the circular accelerator to a location ahead of the near-light-speed particles where electric fields "kick" the charged particles back into place. Run 14 will be the first gold-gold run at RHIC in which stochastic cooling is fully implemented to squeeze beams in three directions—horizontally, vertically, and front-to-back—in both RHIC rings.
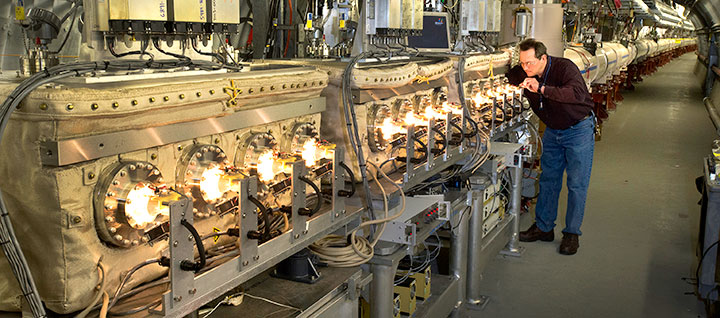
Technician Mike Myers checks components of stochastic cooling "kickers," which generate electric fields to nudge ions in RHIC's gold beams back into tightly packed bunches. This system of squeezing and cooling beams has produced dramatic increases in collision rates—and the data coming out of RHIC.
"We used this system in 2012 with uranium-uranium and copper-gold collisions," Fischer said, "but we've upgraded the longitudinal system for squeezing beams along the direction of the beam since then with improved pickups and kickers to better detect and correct the spread of particles within the ion bunches."
To make even better use of the cooled beams, the RHIC Run Coordinator, Guillaume Robert-Demolaize, has devised a new scheme to make the beams even smaller at the interaction points once their size has been reduced.
Run 14 will also benefit from the first superconducting radiofrequency (RF) cavity installed at RHIC. RF cavities are what accelerate and focus the ion beams, producing strong electromagnetic fields to progressively push the charged particles to higher and higher energy and keep the ions from spreading out too much. Superconducting cavities allow these systems to operate at higher voltage, producing a more powerful push.
"This new RF system provides even more focusing force than the conventional cavities already installed at RHIC," Fischer said. "This will be its first run, mostly focused on commissioning, or testing—but we hope to see some contribution by the end of the run toward improved luminosity."
Even before this run, RHIC was operating at 15 times the luminosity for which it was originally designed. If all goes well, Run 14 could push that number to 18 times the design luminosity, Fischer said.
Detector upgrades
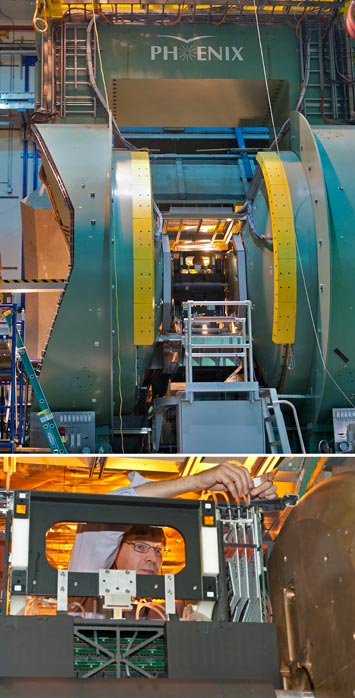
At the heart of the PHENIX detector (top), a silicon vertex tracker (green layers behind the "X" shaped black structure in bottom image) surrounds the point where collisions take place. Together with forward silicon vertex trackers at each end of the barrel (one being adjusted by technician Mike Lenz), these components identify and count the decay products of particles made of heavy quarks streaming out of RHIC collisions.
RHIC's detectors, STAR and PHENIX, are ready to make the most of these exceptionally high collision rates with new capabilities to detect rare particles produced in the plasma. These include particles made of quarks that are heavier than the "up" and "down" quarks found in ordinary matter. Befitting their more exotic nature, they go by the whimsical names of "charm" and "beauty." Particles made of these heavy quarks last for what seems like a fleeting instant, decaying in the time it takes them to travel about 100 microns—about the width of a hair. But even that short flight is long enough for the most sensitive detectors to track and identify these elusive particles through their decay products.
The device installed at STAR for accomplishing this task is called the Heavy Flavor Tracker (HFT). Installed just before the start of the run after many years of development, this $15-million device, built in part at Lawrence Berkeley National Laboratory, is the first silicon detector at a collider that uses Monolithic Active Pixel Sensor technology—"the same technology used in digital cameras," said STAR's Jamie Dunlop, a Brookhaven physicist. "Its silicon sensors have unprecedented thinness—a mere 50 microns, about half the thickness of a human hair. Their thinness and high resolution will allow studies of how particles made of heavy quarks flow from RHIC's quark-gluon plasma."
PHENIX's counterpart is a barrel shaped silicon vertex detector deep within the experiment, with a forward vertex detector at each end of the barrel. "These silicon detectors are incredibly precise and enable us to find particle tracks that don't come directly from the gold-gold collision, but instead originate a few tens of microns away—in other words, tracks of particles likely to have come from the decay of the heavy particles that contain charm or beauty quarks," said Brookhaven's Dave Morrison, a co-spokesperson of the PHENIX collaboration.
"Ensuring that PHENIX takes data successfully requires an enormous, sustained effort across the whole collaboration," Morrison said. The person choreographing that effort for Run-14 is the PHENIX Run Coordinator, Klaus Dehmelt of Stony Brook University, who noted, "With the new silicon detectors, PHENIX now has more than four million electronic channels. It's a very complex and capable experiment and I'm excited to be part of it!"
STAR will also have a fully installed Muon Telescope Detector, built as part of a continuing successful U.S.-China collaboration with additional partners from India. This detector will identify muons, heavier counterparts to electrons, emitted at nearly right angles to the colliding beams, where STAR has already measured electrons. By comparing muon-muon to electron-muon pairs, STAR physicists can separate the production of charm and beauty, as well as various bound states of these heavy quarks, from the shine of electron and muon pairs produced by the high temperature quark-gluon plasma.
This upgrade makes STAR now capable of detecting nearly all the particle types emerging at nearly right angles to the beam, with the exceptions being neutral hadrons, such as neutrons, and neutrinos, which fly through STAR (and almost everything) without interacting at all.
Heavy particles weigh in
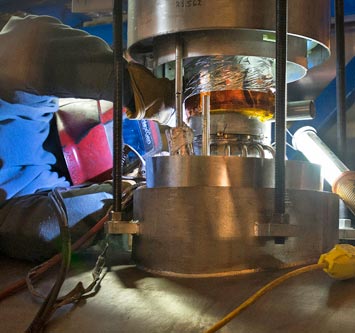
A welder works on the first superconducting radiofrequency cavity installed at RHIC. Superconductivity allows the cavity to operate at higher voltage than conventional cavities, thus producing a stronger focusing force for ion beams. After commissioning, the cavity should further increase RHIC's collision rates.
The improved capabilities at both detectors, along with the increased number of collisions to analyze, will allow measurements of how different kinds of bound states composed of heavy quark-antiquark pairs behave. The most rare of these include J/psi particles (a charm and anticharm quark bound together) and Upsilon particles (a beauty and antibeauty quark bound together).
Both of these bound states are expected to "melt" in the quark-gluon plasma, resulting in lower numbers being detectable than would be without the formation of quark-gluon plasma. Because they melt at different temperatures, miniscule variations in their production can serve as a mini thermometer to more precisely measure the temperature within the seething soup.
Additionally, tracking these rare, heavy particles will offer further insight into the plasma's ability to flow with extremely low viscosity, or resistance—a property that appears to be near zero in RHIC's highest energy collisions, the level considered "perfect" for any fluid.
"I think of the quark-gluon plasma as a flowing stream," said University of Colorado physicist Jamie Nagle, the other co-spokesperson for PHENIX. "If you throw a small pebble into the stream, if the flow is strong enough, the pebble can get caught up in the flow. The charm quark is a middleweight 'pebble' and the beauty quark is a heavyweight (about 3 times heavier than the charm). Comparing how these particles flow can tell you about the strength of the flowing stream and how strongly it grabs onto these heavy quarks—the QGP's 'coupling strength,'" he said.
Measurements of how the heavy particles interact with the plasma will help scientists test early indications that, despite the nearly friction-free environment, some high momentum particles get "stuck" just as those with less energy do. This happens when jets of particles are produced back-to-back near the edge of the interacting matter, with the jet having to traverse a greater distance through the plasma losing more energy than the one with a quick exit. This phenomenon, "jet quenching," was one of the earliest predicted signatures of quark-gluon plasma. Studies of jets made of heavy quarks, with higher momentum—which were expected to make it through the plasma—will test the early counterintuitive observations, and perhaps offer insight into how the plasma stops high momentum particles in their tracks.
A lower-energy investigation
To collect the data relevant to these intriguing questions, physicists will run RHIC at full energy—100 billion electron volts (GeV) per colliding proton or neutron (nucleon)—for 15 weeks. But prior to ramping up to that level, they'll conduct three weeks of collisions at 7.3 GeV per nucleon. That's below the initial energy at which the beams are injected into RHIC, and a testament to the machine's versatility to operate at a wide range of energies.
Why turn down the energy? To explore a gap in data points from RHIC's recent "beam energy scan"—a systematic exploration of how matter transforms from ordinary nuclei to quark-gluon plasma at a wide range of energies and particle densities. That exploration has turned up interesting details about how the behavior of nuclear matter changes above and below the transition, how the type of phase change itself changes at different energy densities, and indicates a possible critical point (where the type-of-transition switch takes place) at right around the missing energy.
"This gap in the energies we've already scanned is right where the data we've collected so far indicates that a few key signatures of the phase transition and a possible critical point may be changing the strongest, and in which interesting behavior may be hiding," STAR's Dunlop said.
Future plans being developed to add an external electron cooling system to the RHIC complex would increase the number of events achievable at low energy by another order of magnitude, producing even more dramatic progress at the low end of the beam energy scan. As part of the plan for that future upgrade, this year's run will incorporate testing of a new magnetic configuration developed by Brookhaven's Christoph Montag, the Low Energy Run Coordinator.
Electron lens testing
Run 14 will also be an opportunity for RHIC's accelerator physicists to test another new piece of recently completed equipment designed to increase luminosity in future proton-proton collisions at RHIC. The devices, called electron lenses, use the attractive force negatively charged electrons have for positively charged protons to compensate for the tendency of the protons in one circulating beam to repel protons in the other.
"There is a repulsive force between the two proton beams, but it is not linear," said Fischer. "It depends on whether the proton is in the middle of the beam or at the edge. This nonlinear force is the dominant source of proton losses in collisions. We want to correct for that with electron lenses."
In this context, the lens is a low-energy electron beam that collides with the proton beam. The electron beam has a current and transverse profile that create the same force for a proton passing through it as the proton experiences when passing through the other proton beam, but with the opposite sign. So the negatively charged beam counteracts the repulsive force experienced by the protons.
"The electron lenses are completely installed, and we can do almost all the testing with gold beams in Run 14," Fischer said. "That way they'll be just about ready to go when we run protons next year."
Leapfrogging technology
"The new technologies being implemented in the accelerator and detectors at RHIC for Run 14 are truly state of the art, in many ways leapfrogging capabilities at Europe's Large Hadron Collider (LHC)," said Mueller. Many of these advances will find application in future upgrades at LHC and elsewhere.
In addition, said Fischer, "while the LHC has higher energy, we will always have more luminosity and greater versatility. And since RHIC is a machine dedicated to the study of heavy ion physics, we also have more time each year to do this kind of science."
This year's run promises to show off these capabilities—and RHIC's continuing leadership in the field.
Research at RHIC is funded primarily by the DOE Office of Science, and also by these agencies and organizations.
DOE's Office of Science is the single largest supporter of basic research in the physical sciences in the United States, and is working to address some of the most pressing challenges of our time. For more information, please visit science.energy.gov.
2014-11606 | INT/EXT | Newsroom