Beam-Beam Compensation Scheme Doubles Proton-Proton Collision Rates at RHIC
Smashing more protons produces more data for exploring physics questions
January 4, 2016
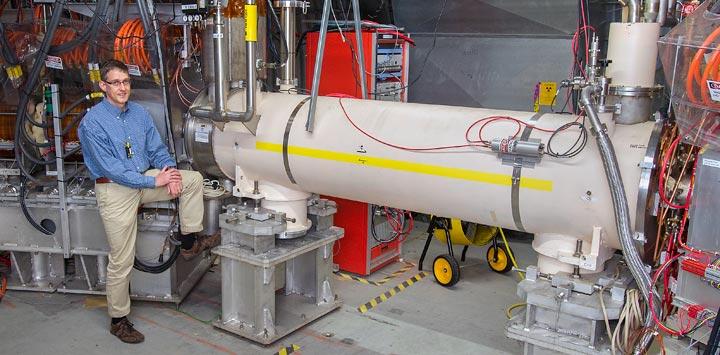
Wolfram Fischer stands next to the electron lensing apparatus at the Relativistic Heavy Ion Collider (RHIC). A schematic diagram showing the components appears below.
UPTON, NY—Accelerator physicists at the U.S. Department of Energy's (DOE) Brookhaven National Laboratory have successfully implemented an innovative scheme for increasing proton collision rates at the Relativistic Heavy Ion Collider (RHIC). More proton collisions at this DOE Office of Science User Facility produce more data for scientists to sift through to answer important nuclear physics questions, including the search for the source of proton spin.
"So far we have doubled the peak and average 'luminosity'—measures that are directly related to the collision rates," said Wolfram Fischer, Associate Chair for Accelerators of Brookhaven's Collider-Accelerator Department and lead author on a paper describing the success just published in Physical Review Letters. And, he says, there's potential for further gains by increasing the number protons from the injectors even more.
Colliding polarized protons
"The strongest disturbance a proton experiences when it travels around the RHIC ring is when it flies through the other proton beam…We've implemented electron lensing technology to compensate for these head-on beam-beam effects."
— Wolfram Fischer, Associate Chair for Accelerators of Brookhaven Lab's Collider-Accelerator Department
RHIC is the world's only polarized proton collider, capable of sending beams of protons around its 2.4-mile-circumference racetrack with their internal magnetic axes (also known as spins) aligned in a chosen direction. Colliding beams of such "spin polarized" protons and manipulating the spin directions gives scientists a way to explore how their internal building blocks, quarks and gluons, contribute to this intrinsic particle property.
Data at RHIC have revealed that both quarks and gluons make substantial contributions to spin, but still not enough to explain the total spin value. More data will help resolve this spin mystery by reducing uncertainties and allowing nuclear physicists to tease out other unaccounted for contributions.
But getting more protons to collide is an ongoing challenge because, as one beam of these positively charged particles passes through the other, the particles' like charges make them want to move away from one another.
"The strongest disturbance a proton experiences when it travels around the RHIC ring is when it flies through the other proton beam," Fischer said. "The result of the positive charges repelling is that the protons get deflecting kicks every time they fly through the oncoming beam."
Opposite charge produces opposite push
The size of the repulsive kick depends on where the proton flies through the beam, with protons about halfway from dead center to the outside edge of the beam's cross-section experiencing the largest outward push. Particles closer to the center or the outer edge of the cross-section experience less repulsion.
Because of the variable shape of this effect—increasing to a peak and then decreasing with distance from the beam's center—it's impossible to correct using magnets. "The magnetic field strength in magnets increases steadily from the center out," Fischer said.
So instead, the scientists turned to using oppositely charged particles to produce a compensating push in the opposite direction.
"We've implemented electron lensing technology to compensate for these head-on beam-beam effects," Fischer said.
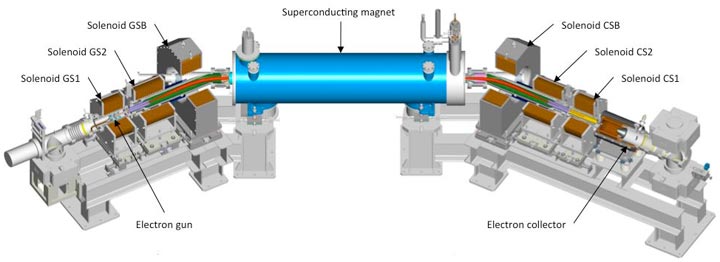
Schematic layout of electron lens components. An electron gun creates a low-energy electron beam that is transported into a superconducting magnet where the magnetic field keeps the electrons from being deflected by the more energetic protons circulating in RHIC. As the protons pass through the negatively charged electron beam, they experience a kick that compensates for the repulsive positive charge of the oncoming proton beam. These kicks nudge the protons toward the center of the beam to maximize proton collision rates.
Essentially, they use an electron gun to introduce a low-energy electron beam into a short stretch of the RHIC accelerator. Within that stretch, the electrons are guided by a magnetic field that keeps them from being deflected by the more energetic protons. As the protons pass through the negatively charged electron beam, they experience a kick in the opposite direction from the repulsive positive charge, which nudges the protons back toward the center of the beam.
"It's not a glass lens like you'd find in a camera," Fischer said, "but we call the technique 'electron lensing' because, like a lens that focuses light, the electron beam changes the trajectory of the protons flying through it."
Riding the optical wave
The scientists also take advantage of certain "optical" properties of RHIC's particle beams to ensure the method's efficacy.
"Ideally you would like to produce these compensating pushes right where the collisions happen, within the STAR and PHENIX detectors," Fischer said. "But then the experiments wouldn't work anymore. So we placed the electron lenses, one on each beam, at a certain distance from the detectors—called the optical distance—where they have an effect at the same point in the 'phase' of the particle beam that's inside the detectors."
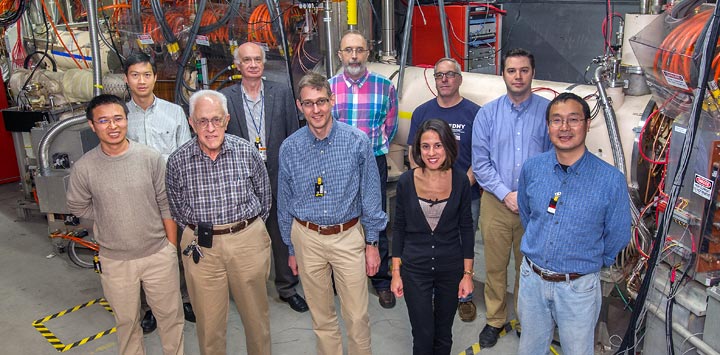
The electron lensing team (front row, left to right): Xiaofeng Gu, Peter Thieberger, Wolfram Fischer, Zeynep Altinbas, Yun Luo, (back, l to r) Chuyu Liu, Alexander Pikin, Al Marusic, Jon Hock, Mike Costanzo. Additional co-authors on the electron lensing paper not shown: Rob Michnoff, Toby Miller, Vincent Schoefer, and Simon White.
Like a wave of light or sound that oscillates up and down in amplitude at a given frequency, the particles that travel around RHIC also oscillate a tiny bit. As long as the nuclear physicists know the frequency of the oscillations and give their electron-lensing kicks at the same point in that oscillation that the particles reach within the detector, the effect will compensate for the proton repulsion the particles experience at that distant location.
So far, the scientists have doubled the proton-proton collision rates at RHIC. They could potentially get even higher gains by increasing the number of protons injected into the machine.
"The key challenge will be to maintain the high degree of polarization the experiments need to explore the question of proton spin," Fischer said. But he insists there is clear potential for even higher proton-proton luminosity.
This work was performed by many people in the Collider-Accelerator Department and the Superconducting Magnet Division at Brookhaven National Laboratory, and was funded by the DOE Office of Science (NP). The scientists also acknowledge the U.S. LHC Accelerator Research Program (LARP) for support of beam-beam simulations, and researchers around the world especially the electron lens experts at Fermi National Accelerator Laboratory.
Brookhaven National Laboratory is supported by the Office of Science of the U.S. Department of Energy. The Office of Science is the single largest supporter of basic research in the physical sciences in the United States, and is working to address some of the most pressing challenges of our time. For more information, please visit science.energy.gov.
2016-11800 | INT/EXT | Newsroom