RHIC Heavy Ions Run-11: a Retrospective
December 5, 2011
By Gregory Marr, Collider-Accelerator Department's Run Coordinator for the Au+Au run in 2011.
Overview
As the heavy ion physics program has evolved at RHIC, improvements and upgrades in the Collider-Accelerator complex have paid off in both peak luminosity and luminosity lifetime. As a result, RHIC has been able to provide sufficient integrated luminosity despite shorter run lengths, as shown in Figure 1. The nucleon-pair luminosity is defined as LNN = A1A2L, where L is the luminosity, and A1 and A2 are the number of nucleons of the ions in the two beams, respectively. In the case of the most recent run (“Run-11” in Fiscal Year 2011), it was a combination of many such improvements that came to fruition in a highly successful, albeit brief, trio of heavy ion programs.
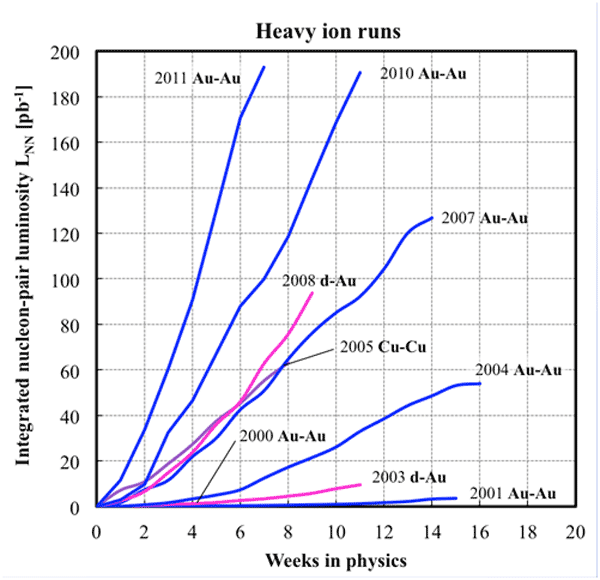
Figure 1: Integrated nucleon-pair luminosity for RHIC heavy ion runs.
Upgrades at RHIC for Run-11
Stochastic Cooling
Stochastic cooling systems, in transverse and longitudinal planes, have been in operation for a number of heavy ion runs thus far. As a result of our prior experience, and complications endured along the way, components were redesigned and repaired during the last shutdown to improve reliability as well as allow for simultaneous cooling of all planes in both rings. A horizontal system was not in place for this run, but the vertical system accomplishes cooling in both transverse planes via coupling. As seen in Figure 2, there is a marked improvement in luminosity lifetime when compared to a store without any stochastic cooling. Emittances are reduced in both Blue and Yellow rings in horizontal and vertical planes, as measured by Ionization Profile Monitors (IPM). Longitudinal emittance is also reduced, as measured by Wall Current Monitors (WCM). IPM and WCM emittances are plotted in Figures 3 & 4 for consecutive stores with stochastic cooling off and on.
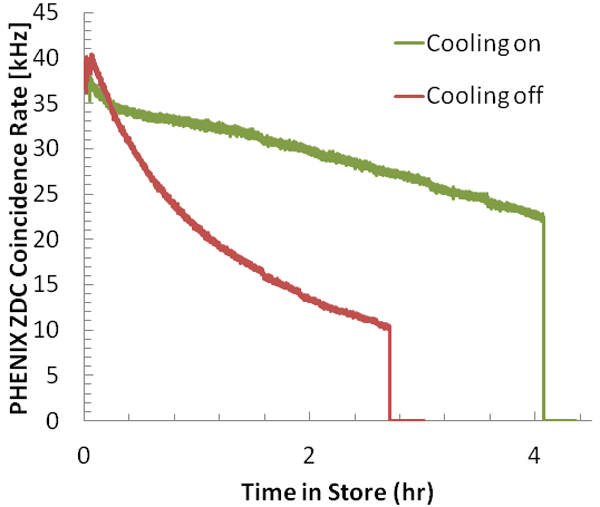
Figure 2: Comparison of luminosity lifetime, for similar store conditions, with and without stochastic cooling, as measured by PHENIX ZDC.
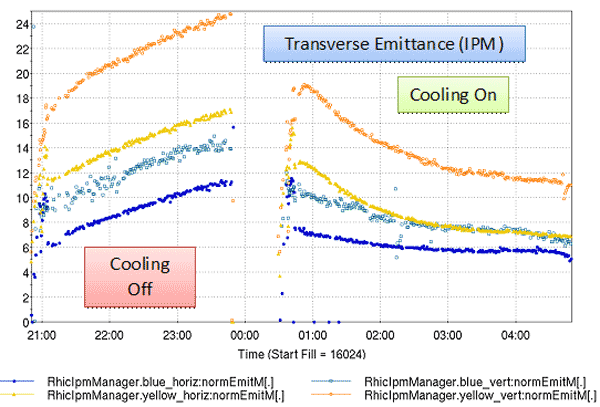
Figure 3: Transverse emittance in Blue and Yellow rings, horizontal and vertical planes, for consecutive stores with cooling turned off and on.
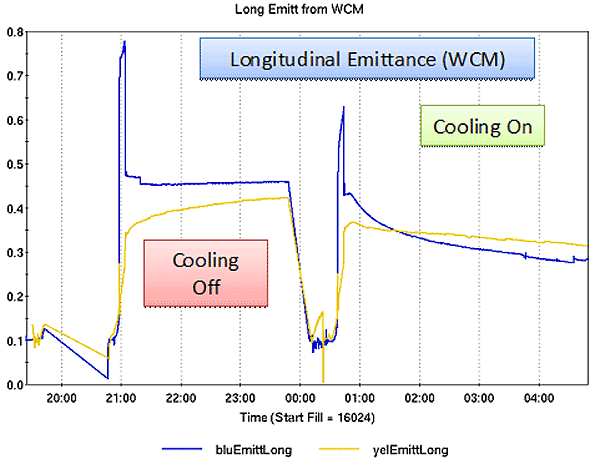
Figure 4: Longitudinal emittance in Blue and Yellow rings for consecutive stores with cooling turned off and on.
Feedback Systems
Feedback systems during the energy ramp to control betatron tune and coupling, as well as orbit, have been utilized during the initial setup period for previous runs, with excellent results – typical measured orbit rms at store is about 20 μm. This year we took advantage of previous efforts and operated feedback systems for all energy ramps over the course of the entire run; additionally, orbit feedback was periodically engaged while colliding at storage energy, in order to maintain a consistent orbit over many hours. Figure 5 compares typical orbit averages and rms deviations during the acceleration ramp, compared to the previous run.
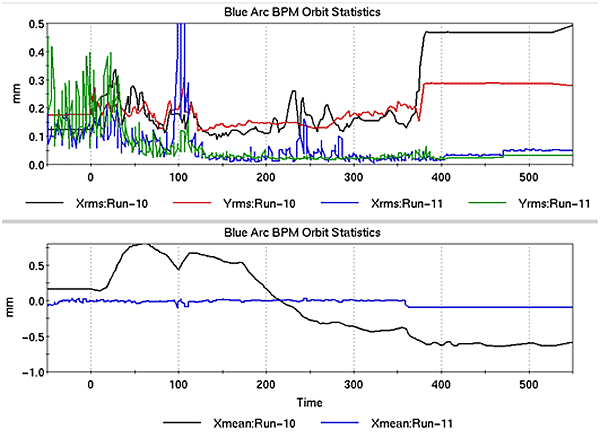
Figure 5: Horizontal and vertical RMS orbit deviations compared to last run (top); average horizontal orbit during acceleration as compared to previous run (bottom).
RF Cavity Relocation
While the 28 MHz, harmonic h = 360 RF acceleration system employs two cavities in each collider ring, the 197 MHz h = 2520 RF storage system, used with colliding beams, previously consisted of three cavities in each ring, with an additional four “common” cavities located at one IP of the Blue and Yellow rings. In previous runs these common cavities posed an intensity limitation due to beam loading from both rings. Prior to this run the common cavities were removed in favor of five storage cavities in each ring.
Beam Dump
Curing intensity limitations would be made moot if one could not safely remove the beam from the machine. Previously at high proton and heavy ion intensities, secondary particles at the beam dump leaked back into the beam pipe and travelled to the superconducting magnet downstream, and could induce a quench. Prior to this year’s runs, inserts were installed in the circulating beam pipe downstream of the dump to shield against the secondaries. No quadrupole quenches were observed in Run-11.
Results
Low Energy Run
Original plans defined the beam energy to be 9 GeV/nucleon. However, RF cavity work during the last shutdown period affected the frequency range of the acceleration cavities. In the course of resolving the issue, the energy was changed to our nominal injection energy of 9.8 GeV/nucleon, which better meshed with the subsequent 100 GeV/nucleon setup and operation. Despite this setback, the collider was in physics production in three days. Figure 6 shows the integrated luminosity for STAR and PHENIX. Nearly all experimental goals were met in 10 days of running. With typical store lengths around 30 minutes, efficient turnaround of stores by operations staff was an integral part of the achieved 71% of calendar time in store. Collimation was used continuously and allowed experimental detector systems to remain on during the refilling process.
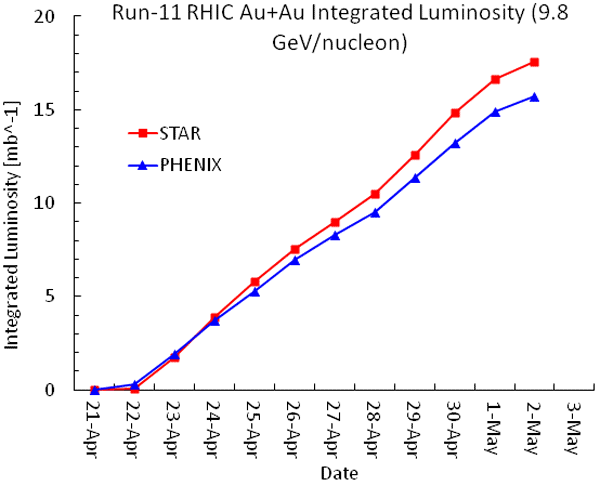
Figure 6: Integrated luminosity over the course of the low energy run, as measured by experiment ZDCs.
High Energy Run
Since the low energy run had already established injection and circulating beam, the 100 GeV/nucleon setup was rapid and completed in 4 days. Beams in both rings were accelerated to full energy on the second ramp attempt, due in large part to the tune, coupling and orbit feedback systems. Figure 7 shows the integrated luminosity for STAR and PHENIX. Included are the conservative (Lmin) and optimistic (Lmax) predictions for the run. Lmin was based upon last year’s performance; Lmax upon projected beam parameters. The achieved luminosity for PHENIX in Run-10 is also displayed for comparison. Since no ramp intensity limitations were observed, high instantaneous luminosities were achieved quickly. In fact, a new record was set for peak instantaneous luminosity in any heavy ion collider; Figure 8 shows the evolution of peak luminosity at RHIC for our gold runs. The initial luminosities at store often exceeded last year’s best values, and played a large role in exceeding the maximum projection for the run.
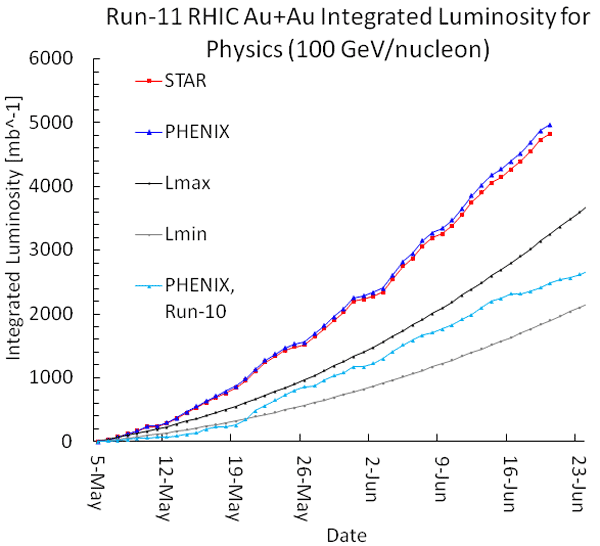
Figure 7: Integrated luminosity for the high energy portion of the run
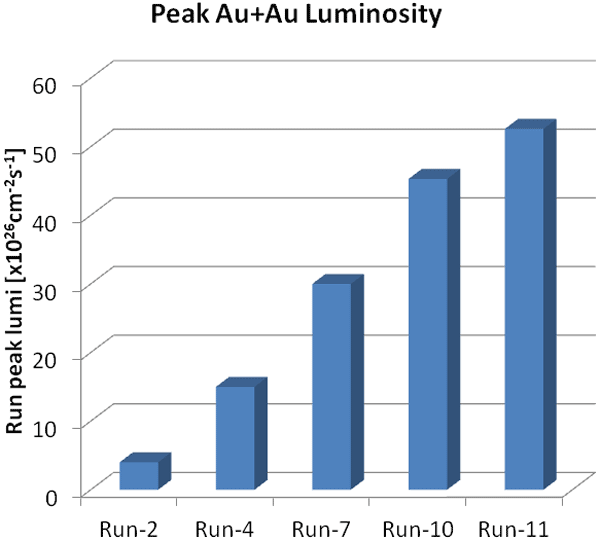
Figure 8: History of peak gold luminosities at RHIC.
Medium Energy Run
Reaching luminosity goals more quickly affords opportunities to include additional programs, such as the 13.5 GeV/nucleon run this year. With beam development previously established for injection, acceleration, and storage at high energy, commissioning of the medium energy was rapid. Beam was accelerated to store in the first attempt, and physics conditions were established in one day. Integrated luminosity, shown in Figure 9, exceeded the experimenters’ goals.
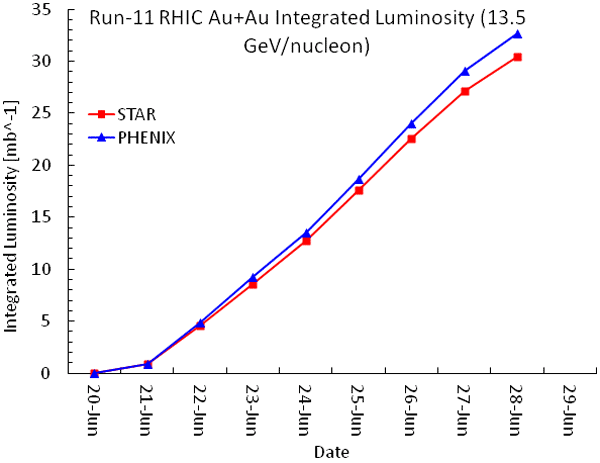
Figure 9: Integrated luminosity for the medium energy portion of the run.
Future Heavy Ion Runs
Thanks to the dedication of operators, accelerator physicists, and support staff, our applied experience from challenges in prior runs paid off with great success for heavy ions in Run-11. For upcoming heavy ion runs, there remain a number of accelerator physics issues that leave room for improvement:
- Stochastic cooling systems for the horizontal plane will soon be made operational.
- The 56 MHz superconducting RF system is under construction.
- Using chromaticity feedback might help in everyday operations.
- Investigation of longitudinal emittance growth along the ramp (which limits the amount of beam in the central 200 MHz bucket at store) will continue.
- Local and global betatron coupling issues remain to be understood.
- Machine lattice development continues in areas such as nonlinearities, beta beating, and near-integer operations.
- Beam intensity limits at gamma transition and during rebucketing need to be rediscovered.
Combined with this, our ability to scan the energy spectrum by setting up different collision energies rapidly, and the advent of new ion choices from EBIS, RHIC can continue to be a powerful, efficient and versatile machine for heavy ion physics.
2011-2756 | INT/EXT | Newsroom