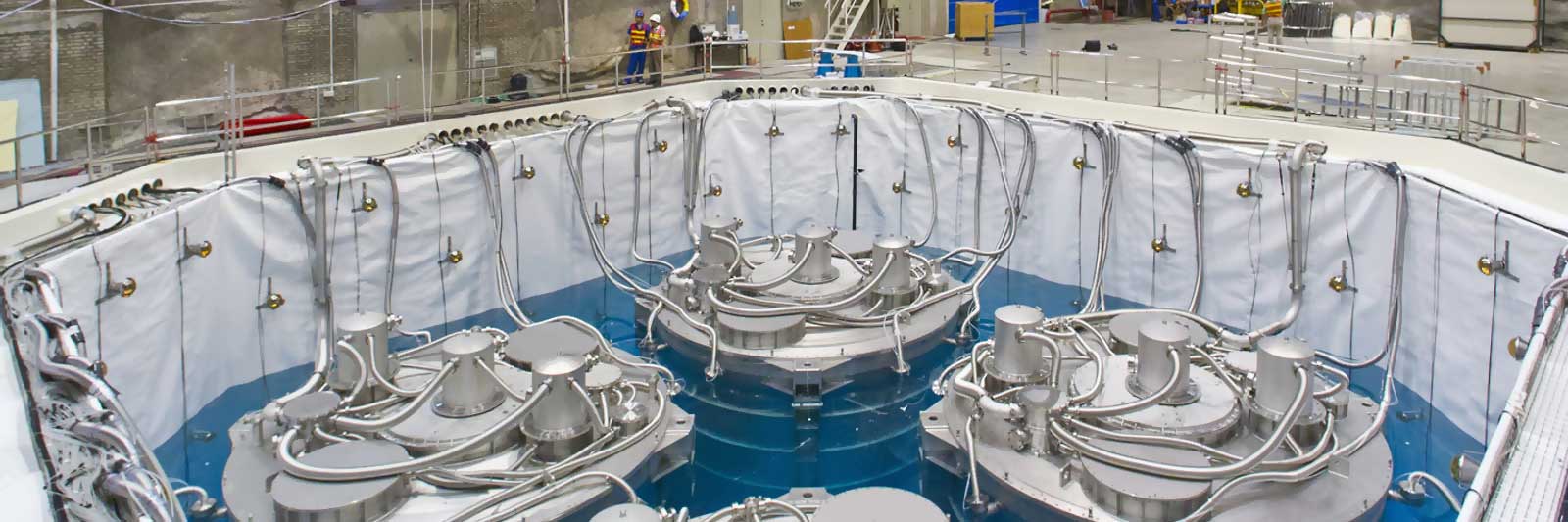
Daya Bay Neutrino Experiment
An international collaboration tracks the safe subatomic send-offs from six nuclear reactors in China to study mysterious particles that may answer questions about the birth of our universe.
Neutrinos—ghostlike particles that flooded the universe just moments after the Big Bang—are born in the hearts of stars and other nuclear reactions. Untouched by electromagnetism and nearly as fast as light, neutrinos pass practically unhindered through everything from planets to people, only rarely responding to the weak nuclear force and the even weaker gravity. In fact, at any given moment, tens of billions of neutrinos are passing through every square centimeter of the Earth’s surface.
To further complicate things, neutrinos come in three fundamental flavors: electron, muon, and tau. The particles actually oscillate between these three forms in a shape-shifting process that only becomes apparent when a given flavor seems to suddenly vanish. The most elusive of these flavor transformations—also called mixing angles—describes the way electron neutrinos transform, and it is essential to understanding the cosmos. The Daya Bay Neutrino Experiment exists to pin that parameter down.
Brookhaven Lab scientists and engineers led the Daya Bay collaboration's detector engineering and design efforts. They perfected the "recipe" for the chemically stable liquid scintillator that fills the experiment's particle detectors where the antineutrinos interact. They also developed software and analysis techniques for measuring neutrino oscillations and play ongoing roles in project management and data analysis.
Detecting the Undetectable
Like all fundamental particles, neutrinos have an antimatter twin. While antineutrinos likely exhibit the same asymmetry found between other matter and antimatter pairs, theory predicts that flavor oscillations are identical. The Daya Bay collaboration needed a powerful antineutrino source and a sophisticated detectors to track the particle transformations.
Liquid Light Traps: Streams of antineutrinos interact with a clear liquid scintillator—a custom chemistry cocktail developed at Brookhaven Lab—inside the detectors and then emit faint flashes of light. Sensitive photomultiplier tubes lining the detector walls then amplify and help record those telltale flashes.
Measuring Disappearance: Daya Bay scientists count the number of electron antineutrinos detected in the halls nearest the reactors and then calculate how many would reach the detectors in the distant hall if there were no oscillation. The number that apparently vanish on the way—actually transforming into other flavors—gives the value of the missing mixing angle.
Antineutrino Producers: The six Guangdong Nuclear Power Group reactors in southern China’s Daya Bay churn out millions of quadrillions of harmless electron antineutrinos every second, giving the collaboration an endless, abundant stream of particles.
Underground Detectors: A series of three experimental halls buried beneath the adjacent hills house a total of six detectors, shielded by the earth from disruptive cosmic rays. The cylindrical antineutrino detectors are also submerged in pools of pure water to protect them from any radioactive decay in the surrounding rock.
A Universe of Matter
The Big Bang should have created matter and antimatter in equal parts, and those particles should have completely annihilated one another, leaving a cosmos filled only with light. But that didn't happen, and the question remains: why does matter exist? The final flavor transformation measured at Daya Bay could reveal that neutrinos and antineutrinos exhibit a dynamic bit of symmetry-breaking known as charge-parity (CP) violation, which may ultimately explain why the Big Bang embraced matter and shunned its anti-twin.
Refined results will influence the design of future neutrino experiments and open the door to further investigations—including how to determine which neutrino flavors are the most massive, whether there is a difference between neutrino and antineutrino oscillations, and, eventually, why there is more matter than antimatter in the universe.