Best Precision Yet for Neutrino Measurements at Daya Bay
By tracking the transformation of neutrinos, scientists hope to answer fundamental physics questions.
September 11, 2015
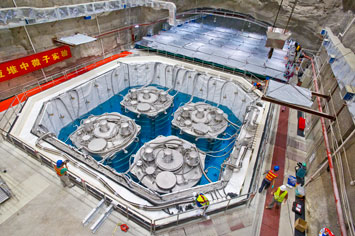
Bird's-eye view of the underground Daya Bay Far Hall during installation. The four antineutrino detectors are immersed in a large pool filled with ultra pure water as a cosmic muon veto system. (Photo by Roy Kaltschmidt, Berkeley Lab)
UPTON, NY—In the Daya Bay region of China, about 55 kilometers northeast of Hong Kong, a research project is underway to study ghostlike, elusive particles called neutrinos. Today, the international Daya Bay Collaboration announces new findings on the measurements of neutrinos, paving the way forward for further neutrino research, and confirming that the Daya Bay neutrino experiment continues to be one to watch.
The latest findings involve measurements that track the way neutrinos change types or flavors as they move, a characteristic called neutrino oscillation. By measuring neutrino oscillation, the researchers can home in on two key neutrino properties: their "mixing angle" and "mass splitting."
Measurements of these properties by the Daya Bay Collaboration are the most precise to date, an improvement of about a factor of two over previous measurements published by the collaboration in early in 2014. The new results will be published in Physical Review Letters.
"We are trying to measure a small effect to a very high precision. Our new result is an important milestone marking the start of the precision era of neutrino physics," said physicist Xin Qian of the U.S. Department of Energy's Brookhaven National Laboratory, which plays multiple roles in this international project, ranging from management to detector engineering and data analysis. The Collaboration includes more than 200 scientists from seven regions and countries.
It's important to measure the mixing angle and mass splitting parameters as precisely as possible, the scientists say, because neutrino behavior could hold the key to understanding the asymmetry between matter and antimatter in the universe. This asymmetry, known as the charge-parity or CP violation, explains why shortly after the Big Bang, when most matter and antimatter annihilated each other, some matter was left over to make up the universe we see today.
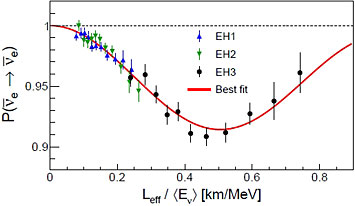
Electron antineutrino survival probability versus the ratio of neutrino propagation distance divided by the energy. The points represent the ratio of the observed number of events divided by the expectation assuming the inverse-square law. A clear deficit is seen and is well described by neutrino oscillation theory (solid line).
The Fluctuating Neutrino
The behavior of neutrinos is unlike any other fundamental particle—they seem to disappear, reappear, and transform themselves as they travel, unimpeded, from sources like the sun and other stars, through space, planets, and even our own bodies.
Neutrinos come in three flavors—electron, muon, and tau. And as a neutrino travels, thanks to quantum mechanical fluctuations, it oscillates between flavors. That is, a particle that starts out as an electron neutrino might at some point turn into a tau neutrino. Then at another point it will present itself more like it did in the beginning. As time goes by, these transformations happen again and again, with the oscillation having a particular amplitude and frequency—similar to sound and light waves.
The amplitude of neutrino oscillations gives scientists information about the rate at which neutrinos transform into different flavors, known as the mixing angle. The frequency of the oscillations gives information about the difference between the masses, a property known as mass splitting.
The Neutrino Net
To study neutrino oscillations, the Daya Bay Collaboration has immersed eight detectors in three large underground pools of water. These detectors sit at different distances from the six China General Nuclear Power Group reactors in Daya Bay. As a by-product of generating electricity, the reactors emit steady streams of electron antineutrinos, which for purposes of the experiment are essentially the same as electron neutrinos. The detectors pick up the transformations that occur as these millions of quadrillions of electron antineutrinos travel farther away from their origin in the reactors.
Our new result is an important milestone marking the start of the precision era of neutrino physics.
— Brookhaven Lab physicist Xin Qian
Based on the data collected over 217 days with six of the Daya Bay detectors and 404 days using all eight of the Daya Bay detectors, the research team has determined the value for a specific mixing angle, called theta13 (pronounced theta-one-three), to a precision two times better than previous results. Similar improvement was made in the precision of measuring the mass splitting.
"We've been able to collect so much data and achieved this level of precision thanks to the spectacular performance of our detectors," said physicist Chao Zhang of Brookhaven Lab. The measurements support the three-neutrino model, which describes physicists' current understanding of the nature of neutrinos, and will have far-reaching implications for future neutrino experiments, he added.
The Daya Bay Collaboration continues to take data. At the end of 2017 it will have roughly four times more data to further improve precision for both the mixing angle of theta13 and the corresponding mass splitting. By then, all three mixing angles and two mass splittings may be determined to comparable precisions, better than three percent, which are essential for future neutrino experiments to measure the remaining unknown properties of the elusive neutrinos.
The unprecedented precision of the data set allows for many other studies: For example, the team is looking for evidence of a possible "sterile" neutrino, a hypothetical type that may mix with the three known neutrino flavors. If this sterile neutrino shows itself in the data, scientists will need to rethink the three-neutrino model. The team is also looking for a variety of other possible deviations from expectations of the Standard Model, the theory physicists use to describe particle interactions.
"By advancing our knowledge about neutrinos, the Daya Bay experiment will expand our understanding of fundamental physics," Zhang said.
Brookhaven Lab's role in the Daya Bay Collaboration is supported by the DOE Office of Science (HEP, NP).
Brookhaven National Laboratory is supported by the Office of Science of the U.S. Department of Energy. The Office of Science is the single largest supporter of basic research in the physical sciences in the United States, and is working to address some of the most pressing challenges of our time. For more information, please visit science.energy.gov.
2015-11747 | INT/EXT | Newsroom