2008 Nobel Prize in Physics Links to Earlier Nobel Work at Brookhaven
October 7, 2008
One American and two Japanese physicists have won the 2008 Nobel Prize in Physics for their work on symmetry breaking. The prize is shared by Yoichiro Nambu of the University of Chicago, Makoto Kobayashi of the High Energy Accelerator Research Organization (Japan), and Toshihide Maskawa of Kyoto University. Nambu will receive half the prize for this work on the general mechanism of spontaneous symmetry breaking, while Kobayashi and Maskawa are being recognized for their theoretical work that ties symmetry breaking to the existence of three families of quarks.
Symmetry plays an important role in physics. The three new physics laureates studied how symmetries are preserved or violated in various ways. Their work builds in part on earlier theoretical and experimental studies at the U.S. Department of Energy's (DOE) Brookhaven National Laboratory, which also received Nobel recognition in 1957 and 1980.
What is symmetry? The geometrical symmetry of the two sides of the human face is an example of something being the same or equivalent. Rotate a sixfold-symmetric snowflake through one-sixth of a circle and it will look the same.
In physics, symmetry is a more general concept and applies to the idea that a physical situation will be the same even if a certain transformation occurs. Three of the most fundamental symmetries bear the name of time reversal invariance (symbolized by the letter T), charge conjugation invariance (C), and parity invariance (P).
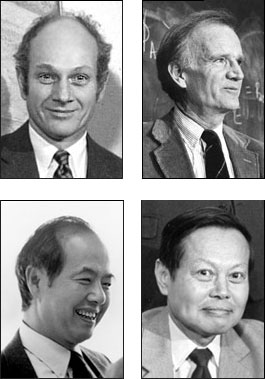
The Brookhaven Connection
In the 1950s, interpreting experiments conducted at Brookhaven Lab, physicists T.D. Lee and C.N. Yang (bottom left and bottom right, respectively) discovered that parity was not invariant. In 1964, also at Brookhaven, James Cronin (top left) and Val Fitch (top right)discovered that the decay of K mesons also violated the combined symmetry of C and P, or CP.
Picture the microscopic interaction of several particles, as if they were billiard balls. Time invariance is the proposition that watching a movie of the interaction would look very like what we'd see if the movie were run in reverse. That is, the interaction is invariant, or the same, even if time is reversed. Charge conjugation refers to the hypothetical replacement of all particles by their antimatter counterparts. Physicists once believed that doing this kind of transformation would leave intact the basic physics of the interaction. Parity invariance is the proposition that if we project all the interacting particles through a hypothetical three-dimensional mirror (replacing all their spatial coordinates (x, y, z) with opposite values (-x, -y, -z) the physics would again appear the same. The universe was, physicists once thought, invariant with respect to all three of these operations.
But nature is complicated. Apparently most symmetries are made to be broken, and that's where the theoretical work of the new Nambu, Kobayahsi, and Maskawa comes into play. How did these asymmetries become apparent? In the 1950s, interpreting experiments conducted at Brookhaven Lab, physicists T.D. Lee and C.N. Yang discovered that parity was not invariant, at least with respect to the weak force. That is, the weak force brought about a particular nuclear decay in which parity was not conserved. Further experiments suggested by Lee and Yang confirmed this work. In 1964, also at Brookhaven, James Cronin and Val Fitch discovered that the decay of K mesons also violated the combined symmetry of C and P, or CP.
These experiments came as something of a surprise, and theorists had to struggle to explain why nature was less symmetric than was thought. The three present Nobel laureates all contributed key papers in this regard.
They addressed the idea that CP violation could account for the fact that in our observable universe matter seems to predominate over anti-matter. The 1972 theory of Kobayashi and Maskawa helped to explain this asymmetry. Furthermore, another implication of their work was the predicted existence of new types of quarks (one of the categories of basic building blocks for matter) beyond those already known. These quarks were later discovered in experiments.
- More information about this year's Nobel Prize
- Background for public (PDF)
- Background for scientists (PDF)
- More information on Lee and Yang's work
- More information on Cronin and Fitch's work
2008-930 | INT/EXT | Newsroom