MicroBooNE Produces Clearest Images of Neutrino Interactions Yet
With updates to its electronics, the state-of-the-art neutrino detector now boasts impressive "signal to noise" sensitivity
August 7, 2017
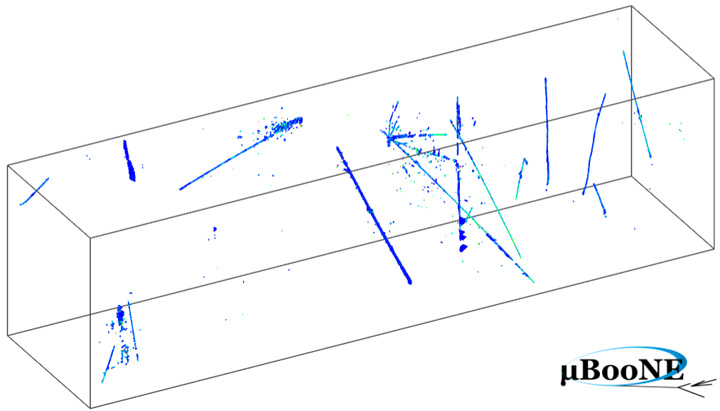
A 3D reconstruction of various particles, including neutrinos, interacting with the argon atoms inside MicroBooNE's time projection chamber (TPC). This reconstruction is based off of when and where electrons produced by such interactions hit the plane of wires at one end of the TPC.
A U.S.-based international collaboration studying “ghost-like” fundamental particles called neutrinos at an experiment known as MicroBooNE has produced the clearest images of neutrino interactions yet. The U.S. Department of Energy’s Brookhaven National Laboratory contributed to the design of this experiment from the beginning, and recently designed novel low-noise “cold electronics” for the detector, which is located at DOE’s Fermi National Accelerator Laboratory (Fermilab). With implementation of sophisticated noise-filtering software and updates to the detector hardware, the MicroBooNE collaboration has produced new clean images that make it easier for researchers to spot and study different types of neutrinos. A paper published in the Journal of Instrumentation illustrates the electronic challenges and solutions that led to this advance.
“These innovations will naturally be included in the next generation of neutrino detector design,” said Brookhaven physicist Xin Qian, the leader of Brookhaven’s MicroBooNE physics group.
The next generation is a big deal, literally: four 17,000-ton neutrino detectors (compared to MicroBooNE’s “small” 170-ton detector) are planned for a future Deep Underground Neutrino Experiment (DUNE). This massive project will attempt to solve some of the biggest mysteries about neutrinos and their role in our universe.
Tracking elusive particles
Trillions of neutrinos—abundant yet elusive particles created in the nuclear reactions powering stars—stream from our sun to Earth every second. But because these particles so rarely interact with matter (which is why we don’t feel them passing through us), the detectors built to spot them must be extremely large and sensitive. To study neutrinos, scientists often also turn to more intense and easily understood sources of these particles: nuclear reactors and particle accelerators. The MicroBooNE collaboration studies neutrinos generated by the Booster proton accelerator at Fermilab, and collects detailed images of their interactions with a detector called a liquid-argon time projection chamber (LArTPC).
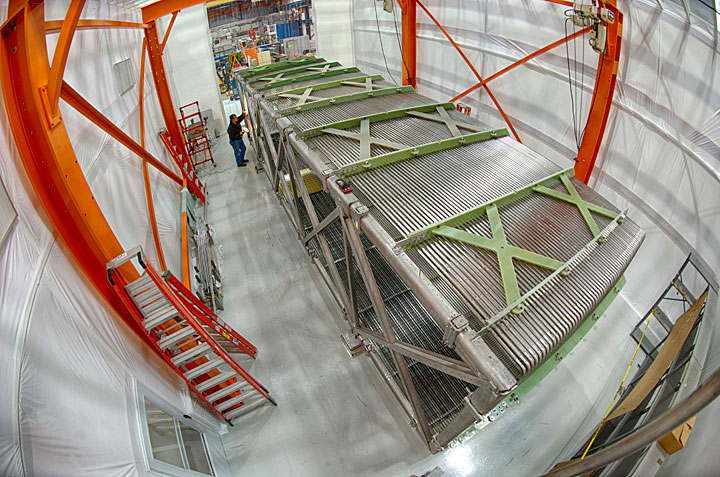
MicroBooNE's time projection chamber—where the neutrino interactions take place—during assembly at Fermilab. The chamber measures ten meters long and two and a half meters high. Photo credit: Fermilab
Although a ‘time projection chamber’ may sound like something from a Michael Crichton novel, it’s a very real technology that has transformed neutrino physics. It’s one of the few types of detectors that can see most of what happens when a neutrino interacts inside.
Neutrinos come in three different “flavors”—electron, muon, and tau. As these neutrinos sail through the LArTPC’s school bus-sized tank of argon, kept liquid at a biting -303 degrees Fahrenheit, they occasionally interact with one of the argon atoms. This interaction produces charged and neutral particles, with a charged particle sometimes corresponding to the type of neutrino involved. The charged particles shoot through the bath, kicking electrons off the argon atoms they pass. These electrons get caught in the tank’s strong electric field and zip toward one end, eventually striking an array of wires. Based on the time and placement of each signal generated when an electron strikes a wire, scientists can figure out where the neutrino collision took place and what it looked like, allowing them to determine the type and energy of the neutrino detected.
Trouble arises, however, when the little currents produced by the kicked-off electrons are muffled by electronic “noise.” Much like static on a radio, noise can drown out the signals of a neutrino collision, making the reconstructed paths blurry and difficult to analyze. According to Jyoti Joshi, a Brookhaven Lab post-doctoral fellow and the leader of the MicroBooNE detector physics working group, the challenge with LArTPC electronics is that “the signal we’re dealing with is so small that we need a very, very sensitive detector to amplify the signal so we can see it. But then, of course, you amplify anything, including noise.”
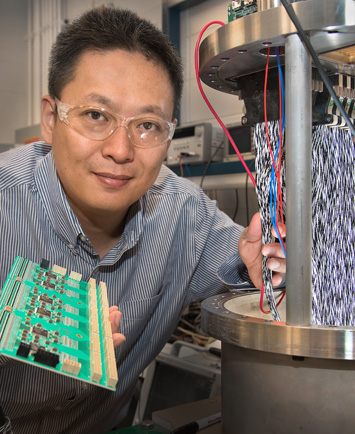
Brookhaven Lab physicist Hucheng Chen holding a replica of one of the 50 cold electronics boards installed in MicroBooNE. He is standing next to a mock-up of one of MicroBooNE's 11 signal feedthroughs—the part of the detector where electronic signals from the cold electronics of the time projection chamber are carried to the warm electronics outside the cryostat.
To try to minimize noise, MicroBooNE researchers worked with the engineers and scientists at Fermilab and in Brookhaven Lab’s Instrumentation Division who had pioneered the development of “cold electronics” for the experiment. Placing the electronics inside the detector tank reduces noise by shortening the path each signal has to travel before getting amplified. But because the tank is filled with liquid argon, these electronics had to be designed to thrive at temperatures hundreds of degrees below zero, long past the range where conventional electronics, like those in your smartphone, can function.
The researchers expected the cold electronics installed at MicroBooNE to produce relatively clean signals and a good picture of the neutrino collisions. But “there are always some surprises,” said Mary Bishai, a senior physicist at Brookhaven Lab. “We had all this excess noise, and at the beginning people blamed the newest technology, the cold electronics.”
After a year of collecting data, the researchers had enough information to pinpoint three sources of excess noise.
“The noise was nearly all from the conventional electronics outside the argon tank,” said Mike Mooney, a Brookhaven Lab post-doctoral fellow and a key contributor in the effort to identify sources of noise.
Most of the noise came from the external power supply for the electronics inside the bath, and from small fluctuations in the high voltage that creates the tank’s electric field. The third and least significant source of noise was an unusual burst that appeared only at a certain frequency, but the team has yet to determine where this final source comes from.
The collaboration initially reduced the excess noise by developing a software program to sift out the desired electron signals. This initial solution allowed them to collect higher-quality data while addressing the actual sources of noise. "We demonstrated that software could remove certain types of noise from the data without losing the very small signals we want to see" said Brian Kirby, the BNL post-doc leading the evaluation of the software fix.
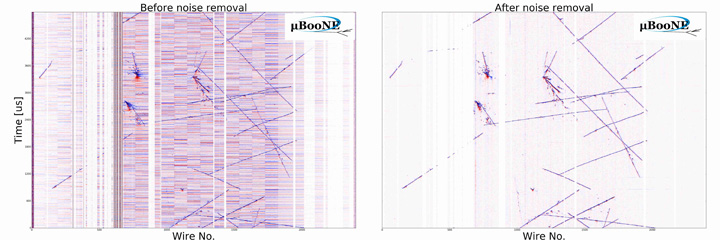
A comparison of particle interaction signals before and after MicroBooNE researchers removed the excess noise.
With the software in place, the researchers could make the necessary changes to the detector’s hardware. They tackled the power supply noise by replacing the part that, just like your laptop charger, converts a higher voltage to a stable lower voltage that the cold electronics require. To combat the noise associated with generating the tank’s electric field, the researchers added a filter that would stabilize the high voltage. They eliminated more than eighty percent of the original noise with these hardware changes alone, and reduced it even further by then reapplying the software filters.
The reconstructed neutrino paths are now sharply clear, like the burst of a small firework that was previously obscured by fog. These clean tracks are absolutely vital as the MicroBooNE team is implementing pattern recognition software to “train” a computer to pick out different types of neutrino collisions.
“This is a really big deal in terms of pushing the field forward,” says Qian. “The lessons we learned will feed back to the next generation of technology development. For this kind of technology, there’s no way we can do it ‘just right’ the first time. We need to try it and improve it, try it and improve it.”
The MicroBooNE collaboration will continue doing just that, trying and improving, as it lays the groundwork for DUNE, the biggest neutrino experiment ever attempted.
Brookhaven’s work on MicroBooNE was funded by the DOE Office of Science (HEP) and the National Science Foundation.
Brookhaven National Laboratory is supported by the Office of Science of the U.S. Department of Energy. The Office of Science is the single largest supporter of basic research in the physical sciences in the United States, and is working to address some of the most pressing challenges of our time. For more information, please visit science.energy.gov.
Follow @BrookhavenLab on Twitter or find us on Facebook.
2017-12311 | INT/EXT | Newsroom