Small Accelerator Promises Big Returns
Under construction in the US, the CBETA multi-turn energy-recovery linac will pave the way for accelerators that combine the best of linear and circular machines
March 16, 2018
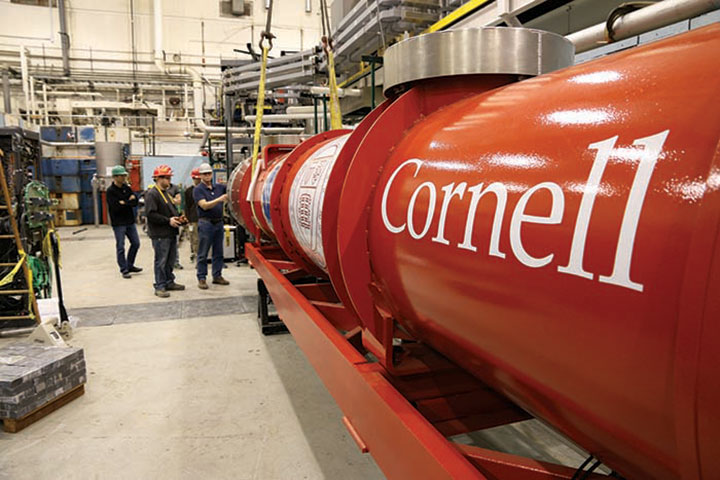
The main linac cryomodule.
Editor's note: This article by Georg Hoffstaetter and Rick Ryan of Cornell University originally appeared in the CERN Courier. For more information about Brookhaven Lab’s role in this work, contact: Karen McNulty Walsh, kmcnulty@bnl.gov, 631-344-8350.
When deciding on the shape of a particle accelerator, physicists face a simple choice: a ring of some sort, or a straight line? This is about more than aesthetics, of course. It depends on which application the accelerator is to be used for: high-energy physics, advanced light sources, medical or numerous others.
Linear accelerators (linacs) can have denser bunches than their circular counterparts, and are widely used for research. However, for both high-energy physics collider experiments and light sources, linacs can be exceedingly power-hungry because the beam is essentially discarded after each use. This forces linacs to operate at an extremely low current compared to ring accelerators, which in turn limits the data rate (or luminosity) delivered to an experiment. On the other hand, in a collider ring there is a limit to the focusing of the bunches at an interaction point as each bunch has to survive the potentially disruptive collision process on each of millions of turns. Bunches from a linac have to collide only once and can, therefore, be focused to aggressively collide at a higher luminosity.
Linacs could outperform circular machines for light-source and collider applications, but only if they can be operated with higher currents by not discarding the energy of the spent beam. Energy-recovery linacs (ERLs) fill this need for a new accelerator type with both linac-quality bunches and the large currents more typical of circular accelerators. By recovering the energy of the spent beam through deceleration in superconducting radio-frequency (SRF) cavities, ERLs can recycle that energy to accelerate new bunches, combining the dense beam of a linear accelerator with the high current of a storage ring to achieve significant RF power savings.
A new facility called CBETA (Cornell-Brookhaven ERL Test Accelerator) that combines some of the best traits of linear and circular accelerators has recently entered construction at Cornell University in the US. Set to become the world’s first multi-turn SRF ERL, with a footprint of about 25 × 15 m, CBETA is designed to accelerate an electron beam to an energy of 150 MeV. As an additional innovation, this four-turn ERL relies on only one return loop for its four beam energies, using a single so-called fixed-field alternating-gradient return loop that can accommodate a large range of different electron energies. To further save energy, this single return loop is constructed from permanent Halbach magnets (an arrangement of permanent magnets that augments the magnetic field on the beam side while cancelling the field on the outside).
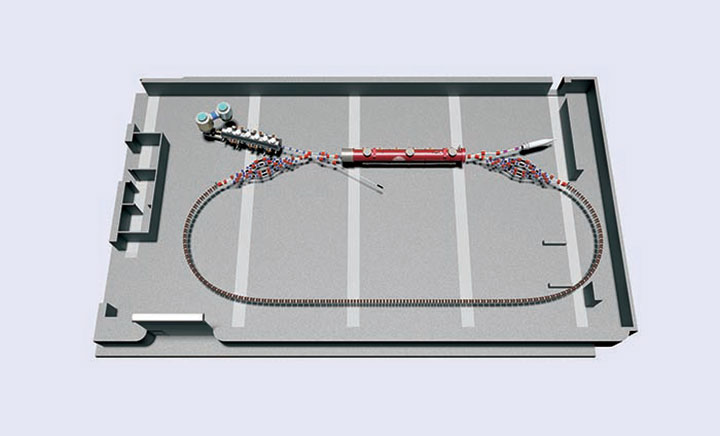
CBETA floor plan.
Initially, CBETA is being built to test the SRF ERL and the single-return-loop concept of permanent magnets for a proposed future electron-ion collider (EIC). Thereafter, CBETA will provide beam for applications such as Compton-backscattered hard X-rays and dark-photon searches. This future ERL technology could be an immensely important tool for researchers who rely on the luminosity of colliders as well as for those that use synchrotron radiation at light sources. ERLs are envisioned for nuclear and elementary particle-physics colliders, as in the proposed eRHIC and LHeC projects, but are also proposed for basic-research coherent X-ray sources, medical applications and industry, for example in lithography sources for the production of yet-smaller computer chips.
The first multi-turn SRF ERL
The theoretical concept of ERLs was introduced long before a functional device could be realized. With the introduction of the CBETA accelerator, scientists are following up on a concept first introduced by physicist Maury Tigner at Cornell in 1965. Similarly, non-scaling fixed-field alternating-gradient optics for beams of largely varying energies were introduced decades ago and will be implemented in an operational accelerator for only the second time with CBETA, after a proof-of-principle test at the EMMA facility at Daresbury Laboratory in the UK, which was commissioned in 2010.
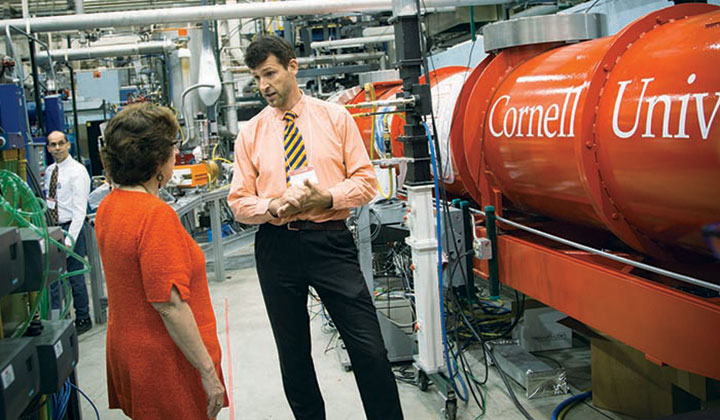
Georg Hoffstaetter and Martha Pollack.
The key behind the CBETA design is to recirculate the beam four times through the SRF cavities, allowing electrons to be accelerated to four very different energies. The beam with the highest energy (150 MeV) will be used for experiments, before being decelerated in the same cavities four times. During deceleration, energy is taken out of the electron beam and is transferred to electromagnetic fields in the cavities, where the recovered energy is then used to accelerate new particles. Reusing the same cavities multiple times significantly reduces the construction and operational costs, and also the overall size of the accelerator.
The energy-saving potential of the CBETA technology cannot be understated, and is a large consideration for the project’s funding agency the New York State Energy Research and Development Authority. By incrementally increasing the energy of the beam through multiple passes in the accelerator section, CBETA can achieve a high-energy beam without a high initial energy at injection – characteristics more commonly found in storage rings. CBETA's use of permanent magnets provides further energy savings. The precise energy savings from CBETA are difficult to estimate at this stage, but the machine is expected to require about a factor of 20 less RF power than a traditional linac. This saving factor would be even larger for future ERLs with higher beam energy.
SRF linacs have been operated in ERL mode before, for example at Jefferson Lab's infrared free-electron laser, where a single-pass energy recovery has reclaimed nearly all of the electron’s energy. CBETA will be the first SRF ERL with more than one turn and is unique in its use of a single return loop for all beams. Simultaneously transporting beam at four very different energies (from 42 to 150 MeV) requires a different bending field strength for each energy. While traditional beamlines are simply unable to keep beams with very different energies on the same "track", the CBETA design relies on fixed-field alternating-gradient optics. To save energy, permanent Halbach magnets containing all four beam energies in a single 70 mm-wide beam pipe were designed and prototyped at Brookhaven National Laboratory (BNL). The special optics for a large energy range had already been proposed in the 1960s, but a modern rediscovery began in 1999 at the POP accelerator at KEK in Japan. This concept has various applications, including medicine, nuclear energy, and in nuclear and particle physics, culminating so far with the construction of CBETA. Important aspects of these optics will be investigated at CBETA, including the following: time-of-flight control, maintenance of performance in the presence of errors, adiabatic transition between curved and straight regions, the creation of insertions that maintain the large energy acceptance, the operation and control of multiple beams in one beam pipe, and harmonic correction of the fields in the permanent magnets.
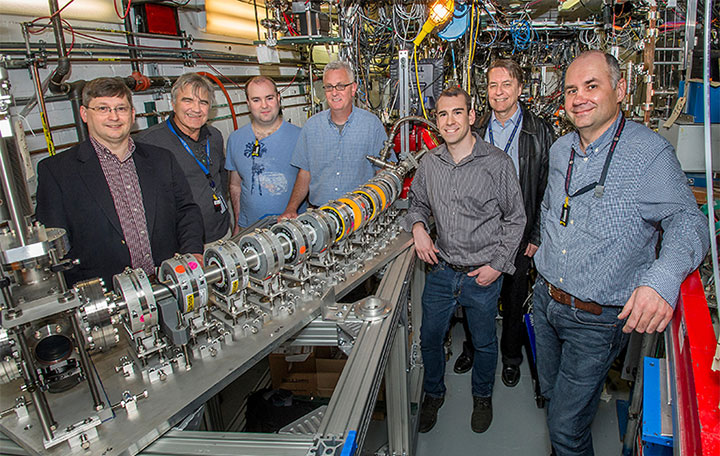
Members of the team testing a fixed-field, alternating-gradient beam transport line made with permanent magnets at Brookhaven Lab's Accelerator Test Facility (ATF), left to right: Mark Palmer (Director of ATF), Dejan Trbojevic, Stephen Brooks, George Mahler, Steven Trabocchi, Thomas Roser, and Mikhail Fedurin (ATF operator and experimental liaison).
Harmonic field correction is achieved by an elegant invention first used in CBETA: in order to overcome the magnetisation errors present in the NdFeB blocks and to produce magnets with 10–3 field accuracy, 32 to 64 iron wires of various lengths are inserted around the magnet bore, with lengths chosen to minimise the lowest 18 multipole harmonics.
A multi-turn test ERL was proposed by Cornell researchers following studies that started in 2005. Cornell was the natural site, given that many of the components needed for such an accelerator had been prototyped by the group there. A collaboration with BNL was formed in the summer of 2014; the test ERL was called CBETA and construction started in November 2016.
CBETA has some quite elaborate accelerator elements. The most complex components already existed before the CBETA collaboration, constructed by Cornell’s ERL group at Wilson Lab: the DC electron source, the SRF injector cryomodule, the main ERL cryomodule, the high-power beam stop, and a diagnostic section to map out six-dimensional phase-space densities. They were designed, constructed and commissioned over a 10-year period and hold several world records in the accelerator community. These components have produced the world’s largest electron current from a photo-emitting source, the largest continuous current in an SRF linac and the largest normalized brightness of an electron bunch.
Setting records
Meanwhile, the DC photoemission electron gun has set a world record for the average current from a photoinjector, demonstrating operation at 350 kV with a continuous current of 75 mA with 1.3 GHz pulse structure. It operates with a KCsSb cathode, which has a typical quantum efficiency of 8% at a wavelength of 527 m and requires a large ceramic insulator and a separate high voltage, high current, power supply to be able to support the high voltage and current. The present version of the Cornell gun has a segmented insulator design with metal guard rings to protect the ceramic insulator from punch-through by field emission, which was the primary limiting factor in previous designs. This gun has been processed up to 425 kV under vacuum, typically operating at 400 kV.
The SRF injector linac, or injector cryomodule (ICM), set new records in current and normalized brightness. It operates with a bunch train containing a series of five two-cell 1.3 GHz SRF cavities, each with twin 50 kW input couplers that receive microwaves from high-power klystrons, and the input power couplers are adjustable to allow impedance matching for a variety of different beam currents. The ICM is capable of a total energy gain of around 15 MeV, although CBETA injects beam at a more modest energy of 6 MeV. The high-current CW main linac cryomodule, meanwhile, has a maximum energy gain of 70 MeV and a beam current of up to 40 mA, and for CBETA will accelerate the beam by 36 MeV on each of the four beam passes.
Several other essential components that have also been commissioned include a high-power beam stop and diagnostics tools for high-current and high-brightness beams, such as a beamline for measuring 6D phase-space densities, a fast wire scanner for beam profiles and beam-loss diagnostics. All these components are now being incorporated in CBETA. While the National Science Foundation provided the bulk funding for the development of all these components, the LCLS-II project contributed funding to investigate the utility of Cornell's ERL technology, and the company ASML contributed funds to test the use of ERL components for an industrial EUV light source.
Complementary development work has been ongoing at BNL, and last summer the BNL team successfully tested a fixed-field alternating-gradient beam transport line at the Accelerator Test Facility. It uses lightweight, 3D-printed frames to hold blocks of permanent magnets and uses the above-mentioned innovative method for fine-tuning the magnetic field to steer multiple beams at different energies through a single beam pipe. With this design, physicists can accelerate particles through multiple stages to higher and higher energies within a single ring of magnets, instead of requiring more than one ring to achieve these energies. The beams reached a top momentum that was more than 3.8 times that of the lowest transferred momentum, which is to be compared to the previous result in EMMA, where the highest momentum was less than twice that of the lowest one. The properties of the permanent Halbach magnets match or even surpass those of electromagnets, which require much more precise engineering and machining to create each individual piece of metal. The success of this proof-of-principle experiment reinforces the CBETA design choices.
The initial mission for CBETA is to prototype components for BNL's proposed version of an EIC called eRHIC, which would be built using the existing Relativistic Heavy Ion Collider infrastructure at BNL. JLAB also has a design for an EIC, which requires an ERL for its electron cooler and therefore also benefits from research at CBETA. Currently, the National Academy of Sciences is studying the scientific potential of an EIC. More than 25 scientists, engineers and technicians are collaborating on CBETA and they are currently running preliminary beam tests, with the expectation of completing CBETA installation by the summer of 2019. Then we will test and complete CBETA commissioning by the spring of 2020, and begin to explore the scientific applications of this new acceleration and energy-saving technique.
2018-12802 | INT/EXT | Newsroom