Argonne Builds on Past Success with Cathode Design for Lithium-Ion Batteries
Design combines the best aspects of different compositions and structures into individual cathode particles
November 1, 2024
By Michael Matz
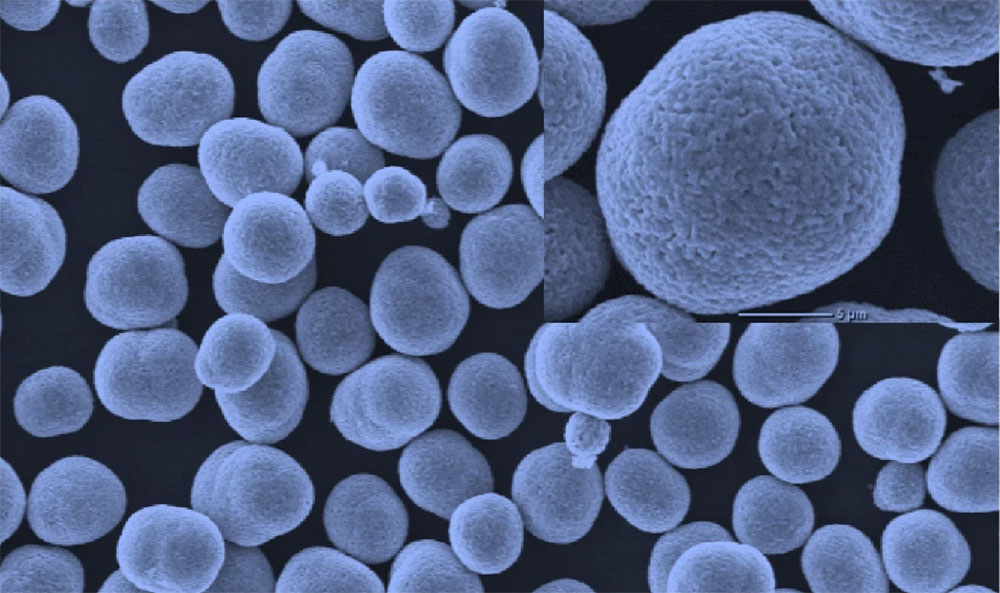
This image, created by a scanning electron microscope, shows the dual-gradient cathode particles fabricated by the Argonne team. (Image by Argonne National Laboratory.)
Editor’s note: The following article was originally issued by the U.S. Department of Energy’s (DOE) Argonne National Laboratory. Researchers used advanced X-ray techniques at DOE Office of Science user facilities, including the National Synchrotron Light Source-II (NSLS-II) at DOE’s Brookhaven National Laboratory. There, they performed full-field transmission X-ray microscopy at the Full Field X-ray Imaging (FXI) beamline, X-ray absorption near-edge structure at the Quick x-ray Absorption and Scattering (QAS) beamline, and 3D nano-fluorescence mapping at the Hard X-ray Nanoprobe (HXN) beamline. This in-depth analysis found that the more disordered surface maintains a lower nickel oxidation state compared to the more ordered bulk, which becomes more highly oxidized during charging. This lower surface oxidation is crucial in preventing unwanted reactions between the electrode material and the electrolyte, which typically leads to degradation. This breakthrough design paves a path forward for next-generation batteries that can deliver both high performance and long-term durability. For more information on Brookhaven’s role in this research, contact Denise Yazak (dyazak@bnl.gov, 631-344-6371).
The U.S. Department of Energy’s (DOE) Argonne National Laboratory has developed a new design that dramatically improves the performance and reduces the costs of lithium-ion batteries.
The design is likely to accelerate adoption of electric vehicles (EVs) and grid energy storage, facilitating global decarbonization efforts. It continues Argonne’s decades-long history of leadership and innovation in battery research.
A dual-gradient design
In 2012, Argonne researchers advanced the state-of-the-art for lithium-ion batteries with a novel cathode (positive electrode) material that significantly increased energy density and durability. The team fine-tuned the composition of nickel, manganese and cobalt in cathode particles to optimally harness the beneficial characteristics of these metals.
“This breakthrough material represents an across-the-board improvement for batteries. It features higher storage capacity, robust stability and heat tolerance at high voltages, and longer lifetimes. Its high energy density enables production of smaller, lower-cost batteries, supporting widespread adoption of EVs and grid batteries.” — Khalil Amine, Argonne Distinguished Fellow and leader of Argonne’s Advanced Battery Technology team
Nickel can increase energy density but also make the surface of the particles too reactive. In Argonne’s design, called a composition gradient, the nickel concentration gradually decreases from the particle core to surface. The idea was to maximize energy density at high-voltage battery operation and minimize reactivity. The high energy density enables the production of smaller, lower-cost batteries.
The cathode particles have a layered structure that is typical of today’s commercial batteries. Ordered layers of metal atoms create pathways to transport lithium ions between a battery’s electrodes. This transport drives the charging and discharging of a battery.
The Argonne design has been patented and licensed to battery and materials manufacturers. Even with the great success of the design, the Argonne team recently began exploring ways to improve it further.
“For EVs to replace gasoline-powered vehicles on a global scale, batteries must be able to operate at higher voltages to deliver even more energy and longer driving ranges,” said Khalil Amine, who leads Argonne’s Advanced Battery Technology team. “At the same time, they must remain safe and cost less to manufacture.”
High-voltage operation tends to cause cathode particles with layered, ordered structures to crack and react more with the battery’s electrolyte. Electrolytes move lithium ions between a battery’s two electrodes, converting stored energy into electricity. This quickly degrades the cathodes, reducing the battery’s capacity and lifetime while increasing safety concerns.
The team’s solution was to add another wrinkle to its composition-gradient cathode design. This involved fabricating cathode particles in which the structure gradually transitions from disordered material on the surface to ordered, layered material in the core.
The particles still possess a concentration gradient involving nickel, manganese and cobalt. The main difference is that the surface is enriched in cobalt and the interior nearly cobalt-free.
The idea behind this approach was to combine the best aspects of different compositions and structures into a single particle. The disordered particle surface would suppress cracking and reactivity while the ordered core would maximize ion transport. In this way, the cathode can potentially achieve high capacity and stability while operating at high voltages.
Demonstrating super-particles
The team performed a series of X-ray, electron and imaging experiments to characterize the new cathode material at rest and while operating. Together, these tests assessed the material at the cathode, particle and atomic levels. The aim was to provide a comprehensive picture of composition, structure and performance. These analyses were carried out at the Advanced Photon Source and Center for Nanoscale Materials at Argonne, as well as the National Synchrotron Light Source II at DOE’s Brookhaven National Laboratory. These are all DOE Office of Science user facilities.
The tests confirmed that the fabrication successfully produced cathode particles with the above structure and composition gradients. Importantly, they showed that the particles remained structurally and chemically stable during high-voltage operation.
“We proved that the disordered particle surface is indestructible, with virtually no reactivity or structural strain,” said Tongchao Liu, an Argonne chemist. Liu is the lead author of the Argonne dual-gradient cathode paper, recently published in Nature Energy. Collaborating partners include DOE’s Brookhaven and Lawrence Berkeley National Laboratory.
The dual-gradient particles were much more durable than the original Argonne design. After charging and discharging the material 500 times, it lost only about 2% of its storage capacity. Based on this finding, the team expects that the material can support a much longer battery lifetime.
The design reduced the overall amount of cobalt in the cathode material. This is important because cobalt is a scarce, costly material and its extraction can have adverse environmental impacts. Composition measurements revealed that most of the cobalt was on the particle surface. The cobalt concentration in the particle’s interior was less than 2% — down from 10-20% in the original design.
“We plan to reduce the cobalt level down to 1%,” said Amine. “This helps us make batteries much more sustainable.”
The team also found that the design enhanced the cathode’s ability to withstand heat. Heat tolerance is crucial to ensure safe operations at high voltage.
Material ready to be licensed
The study marks the first time that composition and structure gradients have been combined in a single cathode particle. It is expected to inspire new lines of research on cathodes that integrate different structures and compositions to enhance battery performance.
“This breakthrough material represents an across-the-board improvement for batteries,” said Amine. “It features higher storage capacity, robust stability and heat tolerance at high voltages, and longer lifetimes. Its high energy density enables production of smaller, lower-cost batteries, supporting widespread adoption of EVs and grid batteries. Our patented design and fabrication process is ready to be licensed by industry.”
This research was supported by DOE’s Vehicle Technologies Office.
In addition to Liu and Amine, authors of the Nature Energy paper include Lei Yu, Junxiang Liu, Alvin Dai, Tao Zhou, Jing Wang, Weiyuan Huang, Luxi Li, Matthew Li, Tianyi Li, Xiaojing Huang, Xianghui Xiao, Mingyuan Ge, Lu Ma, Zengqing Zhuo, Rachid Amine, Yong S. Chu, Wah-Keat Lee and Jianguo Wen.
About Argonne’s Center for Nanoscale Materials
The Center for Nanoscale Materials is one of the five DOE Nanoscale Science Research Centers, premier national user facilities for interdisciplinary research at the nanoscale supported by the DOE Office of Science. Together the NSRCs comprise a suite of complementary facilities that provide researchers with state-of-the-art capabilities to fabricate, process, characterize and model nanoscale materials, and constitute the largest infrastructure investment of the National Nanotechnology Initiative. The NSRCs are located at DOE’s Argonne, Brookhaven, Lawrence Berkeley, Oak Ridge, Sandia and Los Alamos National Laboratories. For more information about the DOE NSRCs, please visit https://science.osti.gov/User-Facilities/User-Facilities-at-a-Glance.
About the Advanced Photon Source
The U. S. Department of Energy Office of Science’s Advanced Photon Source (APS) at Argonne National Laboratory is one of the world’s most productive X-ray light source facilities. The APS provides high-brightness X-ray beams to a diverse community of researchers in materials science, chemistry, condensed matter physics, the life and environmental sciences, and applied research. These X-rays are ideally suited for explorations of materials and biological structures; elemental distribution; chemical, magnetic, electronic states; and a wide range of technologically important engineering systems from batteries to fuel injector sprays, all of which are the foundations of our nation’s economic, technological, and physical well-being. Each year, more than 5,000 researchers use the APS to produce over 2,000 publications detailing impactful discoveries, and solve more vital biological protein structures than users of any other X-ray light source research facility. APS scientists and engineers innovate technology that is at the heart of advancing accelerator and light-source operations. This includes the insertion devices that produce extreme-brightness X-rays prized by researchers, lenses that focus the X-rays down to a few nanometers, instrumentation that maximizes the way the X-rays interact with samples being studied, and software that gathers and manages the massive quantity of data resulting from discovery research at the APS.
This research used resources of the Advanced Photon Source, a U.S. DOE Office of Science User Facility operated for the DOE Office of Science by Argonne National Laboratory under Contract No. DE-AC02-06CH11357.
Argonne National Laboratory seeks solutions to pressing national problems in science and technology by conducting leading-edge basic and applied research in virtually every scientific discipline. Argonne is managed by UChicago Argonne, LLC for the U.S. Department of Energy’s Office of Science.
The U.S. Department of Energy’s Office of Science is the single largest supporter of basic research in the physical sciences in the United States and is working to address some of the most pressing challenges of our time. For more information, visit https://www.energy.gov/science/office-science.
2024-22176 | INT/EXT | Newsroom