Making High-resolution Movies With an Infrared Microscope
November 25, 2013
Infrared beams produced at facilities like the National Synchrotron Light Source represent the lower-energy part of the emitted light spectrum, yet are still much brighter than other sources, allowing scientists to study objects that are just a few micrometers across, like polymer composites, mineral grains, plant-cell walls, microbes, or biological organelles.
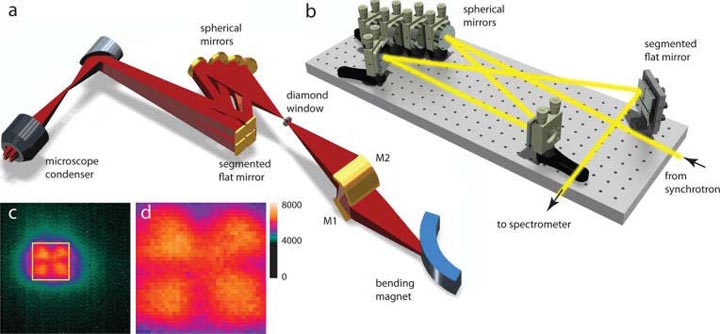
(a) Schematic of the multibeam synchrotron infrared imaging beamline. The bending magnet radiation is extracted and the source is reimaged by means of the elliptical M2 mirror. The diverging fan of light is split into four nearly collimated beamlets, which are subsequently recombined at the sample position. (b) Light path in the optical matching box. Only one beamlet is shown for clarity. Two folding mirrors provide additional control over the beam alignment. (c) Illuminated area of the 128 X 128 pixel focal plane array detector and (d) the enlarged FPA area of 36 X 36 pixels, showing nearly uniform illumination.
Infrared spectroscopic microscopy is a sensitive technique for probing the chemical composition of heterogeneous materials on the micron-size scale. Traditional microscopic measurements with synchrotron infrared light have to be taken spot by spot, sort of like taking a digital picture one pixel at a time.
To speed up the image acquisition time and improve the spatial resolution, the infrared team at Brookhaven National Laboratory developed a method for illuminating a multipixel mid-infrared detector, called a focal plane array, by splitting the infrared beam produced at NSLS into four “beamlets.”
“By splitting the fan of light from a bending magnet at NSLS beamline U10, and carefully manipulating the individual beamlets using a hand-built miniature ‘hall of mirrors,’ we successfully reshaped and steered the beam to illuminate the entire detector without losing its brightness,” said Eli Stavitski, the lead author on the paper.
Taking full images instead of point scanning allows for probing even smaller features, using an approach called oversampling and deconvolution. If the pixels in the detector are small enough, this image reconstruction procedure can be applied to achieve resolution beyond the diffraction limit – potentially down to less than a micron.
“With this enhancement, we were able to identify individual tracks of grey matter in spinal cord tissue samples,” Stavitski said. “The ability to study structure and composition is particularly important since these axonal tracts are affected in neurodegenerative diseases, such as Alzheimer’s disease and ALS, or amyotrophic lateral sclerosis.”
Greater data acquisition speeds also open new avenues for infrared imaging, allowing researchers to record “movies” of chemical transformations where only static “snapshots” were once possible.
These time-lapsed images allowed the Brookhaven team to demonstrate how micrometer-sized polymer beads degrade under ultraviolet radiation, a process that is important in designing both longer-lasting and biodegradable materials. Their method also makes it possible to track biological transformations in living cells as they occur.
With five beamlines dedicated to research with infrared light, NSLS is the most productive synchrotron facility in this field. Though others have demonstrated that marrying a synchrotron source and a focal plane array infrared detector was possible, the solution developed at NSLS allows a relatively straightforward and inexpensive modification of existing beamlines. “Unlike more elaborate designs, this new mirror configuration can be implemented on most infrared beamlines worldwide,” Stavitski said.
This research is described in the paper titled “Dynamic Full-Field Infrared Imaging with Multiple Synchrotron Beams,” published online March 4, 2013, in Analytical Chemistry. The work was supported by the U.S. Department of Energy and the National Institutes of Health.
2013-4445 | INT/EXT | Newsroom