Electrochemical 'Fingers' Unlock Battery's Inner Potential
Scientists track real-time reaction pathways in 3D to uncover new nanoscale clues to increasing lithium-ion battery capacity and optimizing performance
February 27, 2015
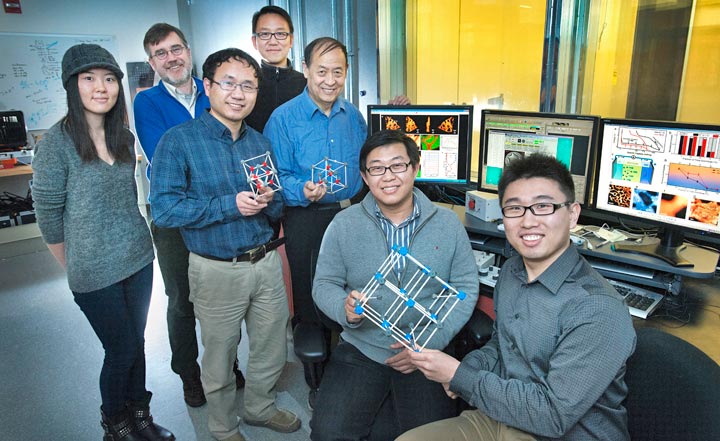
Members of the research team—including (from left) Jing Li, Eric Stach, Xiqian Yu, Dong Su, Xiao-Qing Yang, Kai He, and Huolin Xin—at the Center for Functional Nanomaterials at Brookhaven Lab
Lithium-ion batteries unleash electricity as electrochemical reactions spread through active materials. Manipulating this complex process and driving the reactions into the energy-rich heart of each part of these active materials is crucial to optimizing the power output and ultimate energy capacity of these batteries.
Now, scientists at the U.S. Department of Energy’s (DOE) Brookhaven National Laboratory and collaborating institutes have mapped these atomic-scale reaction pathways and linked them to the battery’s rate of discharge.
Contrary to expectations, a slow discharge rate allows for electrochemical “fingers” to penetrate the electrode material and pry free its stored energy through a process called lithiation. During high-rate discharges, however, these lithiation fingers slowly penetrate layer-by-layer in a much more inefficient fashion.
“This model system reveals the crucial interplay between discharge rate and the lithiation pattern,” said Dong Su, who led the research at Brookhaven Lab’s Center for Functional Nanomaterials (CFN). “The subtle patterns we see can help us develop superior battery architectures that accelerate lithiation penetration and improve overall performance.”
The work was carried out at Brookhaven Lab’s CFN and National Synchrotron Light Source, and SLAC National Accelerator Laboratory’s Stanford Synchrotron Radiation Light Source—all DOE Office of Science User Facilities. The study, published on January 29, 2015, in the journal Nano Letters, includes collaborators from Cornell University, Colorado School of Mines, Lawrence Berkeley National Laboratory, Stony Brook University, and Massachusetts Institute of Technology.
“The initial use of these nickel-oxide electrode materials to store and discharge energy actually helps determine the material’s future performance,” said Kai He, the first author and a post-doctoral fellow working at the CFN. “The discharge process starts near the surface, and then moves into the interior through ‘fingers’ to unlock the material’s full capacity. The neat thing is that we were able see this transition happening for the first time.”
“Creeping” fingers
Six seconds into this video clip, see the "lithiation fingers" penetrate the nickel-oxide electrode material as they pry free stored energy and the battery discharges. The video shows the process 30 times faster than real time and was produced with a transmission electron microscope at the Center for Functional Nanomaterials at Brookhaven Lab.
The study showed that several different reaction pathways can occur in these materials. Although the surface reactions move rapidly, they do not penetrate deeply into the material, so this reaction pathway can contribute only a small amount to the overall energy capacity and output of the battery.
“Over time, the near-surface reaction propagates uniformly from the outer surface of each nanoparticle inward from all directions—this is what we call the shrinking-core mode—but it can be exceptionally slow,” He said. “That inner capacity remains mostly untouched until the lithiation fingers form.”
These nucleation fingers then spread much like tree roots creeping through energy-packed soil, unlocking electricity as they go.
“We expected this process to occur much faster during high-rate discharges, but we found the opposite to be true,” Su said. “High rates spread across the material’s surface, but then become abruptly stalled. At slow rates of discharge, however, the penetrative fingers quickly formed and opened the way for steady, high-capacity use.”
The lithiation fingers—the key to unlocking full capacity—require a fixed incubation time to form and grow, which sets up a timescale limit for efficient, high-speed energy storage in lithium ion batteries.
Said study coauthor Feng Lin, a materials scientist at the Lawrence Berkeley National Laboratory, “We chose to perform our study using uniquely designed nickel oxide nanoscale materials. These materials are somewhat two-dimensional, and provide clear-cut crystal orientations for experimental observation and theoretical modeling. We expect that similar phenomena are applicable to other related electrode materials.”
X-ray and electron probes
The collaboration combined data from electron microscopy, x-ray spectroscopy, and computational modeling.
“We used beams of electrons focused to a size of 1 Angstrom (10-10 meters) to map the physical paths of these reactions,” said Eric Stach, who coauthored this paper and leads the CFN’s electron microscopy group. “Using transmission electron microscopy, we made real-time movies of the the lithiation patterns. This enabled us to directly visualize how this process occurs at the nanoscale. We also characterized the lithium-ion samples after discharge to further map the structural and chemical changes with three-dimensional electron tomography.”
This data was corroborated by x-ray spectroscopy studies conducted at Brookhaven’s National Synchrotron Light Source and SLAC Lab’s Stanford Synchrotron Radiation Light Source.
The x-ray studies quantitatively tracked the chemical changes within the samples, revealing the rates of lithiation reactions and the evolving chemical structure. Computational models then helped interpret this data and explain the electrochemical link between discharge rate and reaction propagation.
“The range of talent, expertise, and instruments from labs across the country lets us get a complete portrait of the entire reaction at all relevant length scales, linking electrochemistry and reaction mechanism with nanostructures,” said He.
The researchers plan to apply this same methodology to more systems and expand its use as a guide for new, high-performing battery engineering.
This work was supported by the DOE Office of Science (BES), the DOE Office of Energy Efficiency and Renewable Energy, and the National Science Foundation.
Brookhaven National Laboratory is supported by the Office of Science of the U.S. Department of Energy. The Office of Science is the single largest supporter of basic research in the physical sciences in the United States, and is working to address some of the most pressing challenges of our time. For more information, please visit science.energy.gov.
2015-5481 | INT/EXT | Newsroom