Producing Cold Electron Beams to Increase Collision Rates at the Relativistic Heavy Ion Collider
Engineers and physicists team up on new technology for keeping particle beams tightly packed, with possible applications at a future electron ion collider and other facilities
December 7, 2015
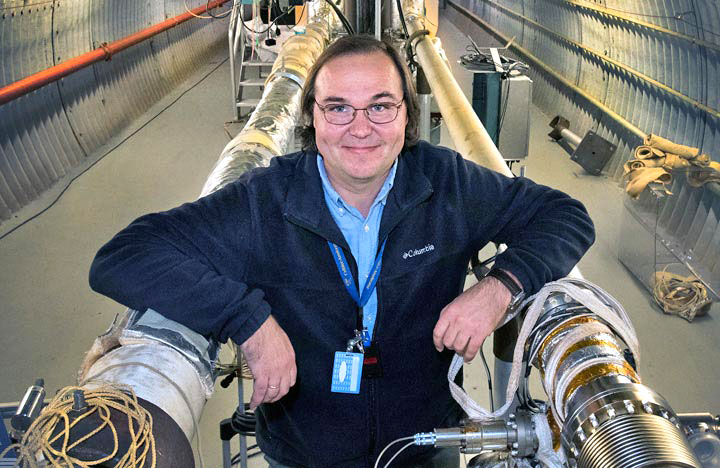
Brookhaven Lab accelerator physicist Alexei Fedotov standing between side-by-side beampipes of the Relativistic Heavy Ion Collider (RHIC) in a section where physicists plan to introduce electrons to cool the ion beams. Ions traveling in opposite directions cross and collide at interaction points to recreate the conditions of the early universe. Electron cooling will maximize collision rates, generating more data for discoveries.
Accelerated ion beams heat up. As the particles that make up the beam move around and scatter off of one another, some speed up while others slow down, leading to a wide spread in their velocities. This spreading of velocities heats and enlarges the beam, which creates a challenge for physicists trying to get the particles to collide. So physicists at the Relativistic Heavy Ion Collider (RHIC), a nuclear physics research facility at the U.S. Department of Energy’s Brookhaven National Laboratory, are exploring ways to cool the beams and keep their particles tightly packed.
“This will be the first time electron bunches produced by a photoemission electron gun are used for beam cooling at an operating facility.”
— Brookhaven Lab accelerator physicist Alexei Fedotov
“You would like to get as small a temperature in the beam as possible,” said Alexei Fedotov, a Brookhaven Lab accelerator physicist working at RHIC. A low temperature means tightly packed particles and an increased likelihood the ions will collide.
RHIC, a DOE Office of Science User Facility, is all about producing these subatomic particle smashups. Its collisions of heavy ions such as gold nuclei have given scientists their first glimpse of the building blocks of matter—quarks and gluons—as they existed just microseconds after the Big Bang. They’ve also revealed details about the strong nuclear force that holds these building blocks together to form nearly all the visible mass in the universe today.
One of RHIC’s most profound discoveries has been the “perfect liquid” nature of the free-flowing plasma of quarks and gluons that filled the early universe. By embarking on a detailed study of this quark-gluon plasma (QGP), scientists hope to learn more about how the universe evolved from a primordial soup to a place filled with planets, stars, and wonder.
Getting high collision rates at low energy
As part of that goal, RHIC physicists have been colliding beams over a wide range of energies to explore the nuclear “phase diagram”—a map of the characteristics of nuclear matter over various conditions of temperature and density. They’re looking for evidence of phase changes—like those that transform liquid water to steam and ice—and also a possible critical point, where the type of transition from ordinary nuclear matter to QGP itself changes from a smooth crossover to a sudden transition, like water boiling in a pot.
RHIC has lots of data from collisions at very high energies. But to plot certain points on the nuclear phase map, RHIC physicists have to turn the collision energy down.
“RHIC can operate at lower energy to explore this part of the phase diagram. But unfortunately, the temperature of the beams at these energies is strongly increased due to the particles scattering off one another,” Fedotov said. That means the rate of collisions, or luminosity, is too low to get adequate data. “This is why we want to look for ways to cool the beams—to increase luminosity,” Fedotov said.
Using cold electrons to cool ions
The idea of using an electron beam to extract heat from RHIC’s hot ions seems at first fairly straightforward: “If you have something hot, you want to put something cold in the same volume so they come to some medium temperature,” Fedotov said. The temperature of a particle beam is proportional to the particles’ mass and the spread of beam velocities, so beams made up of low-mass electrons have an inherently cooler temperature than heavy ion beams, as long as the two are moving at the same speed.
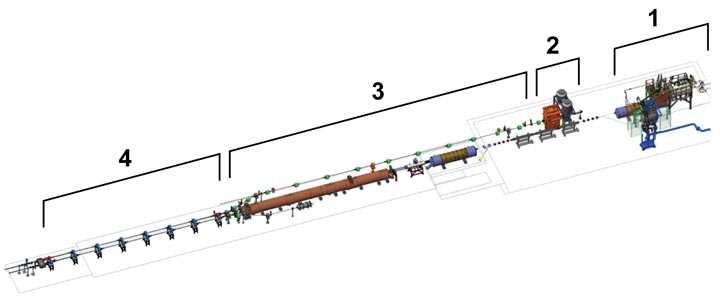
A schematic of low-energy electron cooling at RHIC, from right: 1) a section of the existing accelerator that houses the beam pipe carrying heavy ion beams in opposite directions; 2) the direct current (DC) electron gun and other components that will produce and accelerate the bright beams of electrons; 3) the line that will transport and inject cool electrons into the ion beams; and 4) the cooling sections where ions will mix and scatter with electrons, giving up some of their heat, thus leaving the ion beam cooler and more tightly packed.
In fact, physicists have been using this “electron cooling” technique to keep relatively low-energy particle beams cool since the 1970s. They use an electrostatic generator to create a continuous beam of cool electrons and inject that beam into the flow of ions. As the hot ions scatter off the cold electrons, they give up some of their energy—their heat.
This method could work for cooling RHIC beams at low energy. But eventually the scientists would like to use electrons to cool RHIC beams accelerated to billions of electron volts—both for future studies at RHIC and for achieving the high luminosity required for a proposed future electron ion collider (EIC). The trouble is getting electrons up to that energy.
“If you have a train moving at high speed and your electrons are moving at slow car speed, they cannot cool the ions on the train since they cannot catch up with the train,” Fedotov said. “You have to accelerate electrons to the same speed as ions so they move together for this technique to work.”
So RHIC physicists will use a brand new electron cooling approach based on linear radiofrequency (RF) acceleration of electron beams during the next beam energy scan, with installation of the first cooling section elements to start this December. New methods of generating high-brightness electron beams would also play a key role in an energy-recovery electron accelerator component of an EIC.
Photocathodes and an electron gun
The scientists’ first step in developing the new technique was to scrap the concept of an electrostatic generator, which, even for low-energy continuous electron beam cooling, would have to be quite large—too big to fit inside the RHIC tunnel. Instead, they’re developing photocathodes to install in a high-current electron gun. This gun will produce extremely bright bunches of electrons (rather than a continuous stream) and accelerate them to meet up with and cool the ion bunches at RHIC.
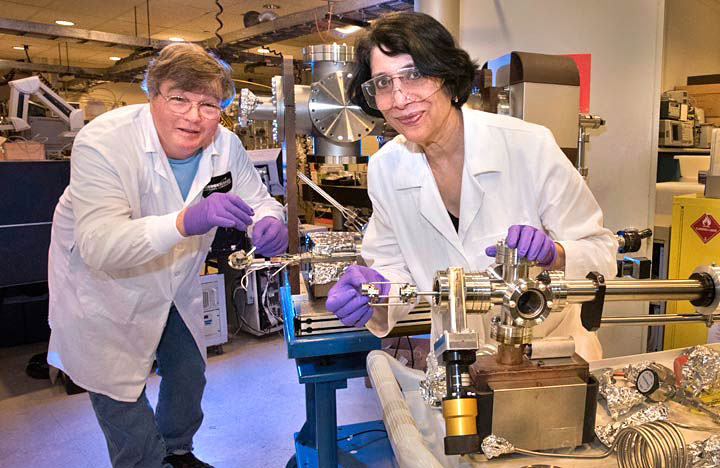
John Walsh of Brookhaven's Instrumentation Division adjusts a photocathode assembly for the apparatus the team is using to test the cathode preparation process, while Triveni Rao loads sample photocathode discs into a "suitcase" chamber for transporting them. The discs for low-energy electron cooling will be somewhat larger and are extremely sensitive to oxygen and moisture. So the chambers that make up the production level system (schematic below) will be pumped to maintain ultra-high vacuum conditions throughout cathode fabrication and transportation to RHIC.
Physicists and engineers in Brookhaven Lab’s Instrumentation Division have been working for many years on ways to generate high-brightness electron beams for research experiments. Their methods take advantage of the photoelectric effect—the tendency of materials to emit electrons when struck by light of an appropriate wavelength.
“In an electron gun, there’s a cathode and anode. When a voltage is applied to one of them, it sets up an electric field between them. Part of the cathode is coated with a photo-emissive material, which means when you shine a laser on it, the photoelectric effect makes electrons come out. When that happens, the electric field accelerates the electrons,” explained Instrumentation Division physicist Triveni Rao. “If you accelerate the electrons quickly, you can keep the brightness high.”
But even state-of-the-art RF guns, conventional sources of high-brightness electron beams, produce a fairly low current—about 120 billionths of an amp.
“For low-energy electron cooling at RHIC we need to generate nearly a million times more current, about 50 milliamps, to match the rate of the ion bunches we are trying to cool. And for other future applications, like an energy-recovery electron accelerator we are developing for an electron ion collider, we’ll need tens of millions of pulses per second,” Rao said. “Although higher-current RF guns are in development, none is operational in a facility yet. So we have to rethink the whole concept—the cathode, the gun, and how we’re going to handle the beam,” she said.
The number of electrons emitted by a photocathode depends on the wavelength of the laser. For example, a typical metal photocathode requires a laser wavelength of approximately 250 nanometers. Such short-wavelength lasers with sufficient energy are hard to produce and don’t yet exist. So Rao’s group is trying to amp up the electron output from the other end—by developing photocathodes from materials that let go of their electrons more easily at longer wavelengths.
“Unlike the metal photocathodes used in conventional RF guns, these new photocathode materials are very sensitive to contamination by moisture and exposure to oxygen, so we have to make them in an ultrahigh vacuum,” Rao said. Plus, the materials don’t last as long as bulk metal photocathodes, so they have to be replaced frequently—possibly as often as every one to two days.
Sophisticated evacuated “suitcase”
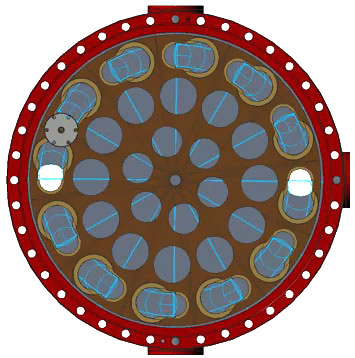
Twelve photocathodes will be loaded into the apertures on the outside edge of this circular "magazine," which will fit inside the "suitcase" that transports them to RHIC and inserts them into the electron gun. The circles closer to the center of the circle are holes to reduce the weight of the device and increase pumping to improve the vacuum in the chamber.
These issues of material sensitivity and degradation are a particular challenge for an operating physics facility like RHIC, which runs continuously—24 hours a day, seven days a week—with the accelerator tunnel completely off limits for several months at a time, except for maintenance periods of about six hours once every two weeks.
“We’re doing lots of experiments to investigate how to make the best photocathodes, how to improve their quantum efficiency—how many electrons you get per photon of light—how they respond to contamination, how many we’ll need, and how quickly we can replace them,” Rao said.
The current scheme calls for making more than a photocathode a day in an ultrahigh vacuum chamber in the Instrumentation Division. Attached to the chamber, like something out of a James Bond movie, will be “the world’s most sophisticated evacuated ‘suitcase’”—a portable apparatus made of pipes, chambers, and valves that will maintain the vacuum and transport the photocathodes, 12 at a time, to the electron gun at RHIC.
“The Collider Accelerator Department will attach this suitcase to equipment in the RHIC vacuum tunnel so the photocathodes can be inserted into the gun while still under ultrahigh vacuum,” Rao said. While one suitcase is attached to RHIC, the physicists in Instrumentation will be making 12 more photocathodes in another, and the two suitcases will shuttle back and forth between the facilities. At RHIC, an automated system that maintains the vacuum will swap out the photocathodes as they degrade while RHIC is running.
“We are planning with very conservative assumptions—replacing the photocathodes every day or two—but they may last longer,” Fedotov said.
For the low-energy proof-of-principle test, the physicists will be using a direct current (DC) electron gun, designed and built by collaborators at Cornell University. Cornell has demonstrated that this DC gun can reach the required 50-milliamp level of current.
“If we can demonstrate that we can make and replace the photocathodes and efficiently cool low-energy ion bunches at RHIC, this will be the first time electron bunches produced by a photoemission electron gun are used for beam cooling at an operating facility,” Fedotov said.
Higher-current electron guns will be required for future projects—things like high-energy electron cooling and building a high-brightness electron accelerator for the proposed EIC. The success of the low-energy electron cooling experiments would lay the foundation for building those future technologies.
This work was supported by the DOE Office of Science (NP).
Brookhaven National Laboratory is supported by the Office of Science of the U.S. Department of Energy. The Office of Science is the single largest supporter of basic research in the physical sciences in the United States, and is working to address some of the most pressing challenges of our time. For more information, please visit science.energy.gov.
2015-5917 | INT/EXT | Newsroom