NSLS-II User Profiles: Wilson Chiu
June 7, 2016
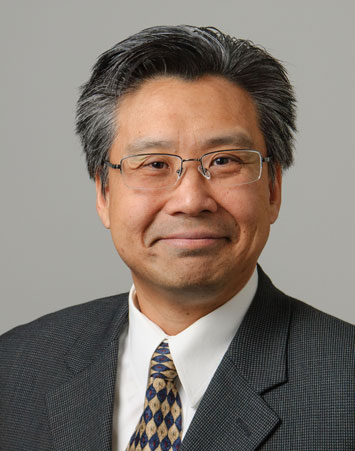
Wilson K. S. Chiu
Wilson K. S. Chiu, a professor in the Mechanical Engineering Department at the University of Connecticut, recently conducted an experiment as one of the first general users at the National Synchrotron Light Source II (NSLS-II) Hard X-Ray Nanoprobe (HXN). He studied “mixed ionic electron conducting” (MIEC) materials, which are engineered materials that can, for example, separate oxygen or other gases from the air or carbon dioxide from the waste stream of a fossil fuel plant.
What is your background and current research interests?
I have been a synchrotron user for more than 10 years. My lab studies materials that can store and convert energy, such as battery and fuel cell materials. These are actually made of small grains of different materials that are interconnected in three dimensions. I am amazed to see how they function to produce electricity. In particular, I am interested in how the 3-D microstructure of fuel cell materials can be engineered to work better.
I started working with Francesco De Carlo at the Advanced Photon Source (APS) at Argonne National Laboratory and Yong Chu, the NSLS-II HXN beamline leader, who was at APS at the time. We wanted to use an x-ray microscope to obtain 3-D images of fuel cell materials that were somewhat like CAT scan images, but with nanometer-scale resolution; we wanted images that could provide details of the materials' 3-D structure and chemical make-up at the nanoscale. Back then, there were very few tools that could provide this kind of information, and those that could weren’t suited to imaging dense materials.
Imaging with x-rays is unlike other types of imaging in that it can penetrate dense materials that visible light can't. X-rays can pick out particular elements and can allow us to study materials as 3-D chemical and microstructural changes are taking place within them. The full-field microscope we used at the APS had an imaging field of view of about 20 microns, yielding images with a spatial resolution of about 30 nanometers. But we wanted to see what was happening at the boundaries between the grains, down to the smallest region of the nanoscale. We were never quite able to get the resolving power and detection sensitivity we needed.
My collaborators and I have been publishing our work in this area, documenting our progress in studying various energy materials, and extending this microscope’s capability for 3-D elemental sensitivity, chemical mapping, and in situ measurements. Since then, Yong Chu has been creating new instruments with better capabilities. When he came to Brookhaven to develop the Hard X-ray Nanoprobe, we continued our collaboration with him and his team.
Please describe your experiment at NSLS-II.
We were studying a mixed ionic electronic conducting (MIEC) material, which is a composite of two materials that have been sintered together yet retain their separate phases. One of the phases is an electronic conductor (conducts electrons) and the other is an ionic conductor (conducts oxygen ions). The particular MIEC we studied is designed for concomitant transport of oxygen ions and electrons. Possible applications are purifying metals and separating gases, and use in energy conversion and storage materials, such as batteries and fuel cells.
At this point, however, our work has focused on basic research and understanding how MIEC materials work. One issue we are looking at is why MIECs sometimes degrade quickly; another challenge is increasing the amount of ions they can transport so that the membrane system can operate more efficiently. Our experiment at the HXN was a fundamental study on the MIEC's properties, and the phases that are present. There is some evidence that emergent phases appear during processing, which could potentially degrade (or enhance!) the MIEC's performance. In the past we have found that there is a “sweet spot” in which the performance increases significantly. At the HXN, we were trying to figure out why. We used x-ray fluorescence (XRF) imaging to study these emerging secondary phases, which exist at the grain and grain boundary. XRF imaging can tell us about the elements that are at these boundaries, which have features of only a few nanometers in size.
Why did you choose NSLS-II for your research?
The HXN at NSLS-II offers the highest spatial resolution in the hard x-ray regime, which is necessary for accessing the key absorption edges of high-atomic-number elements found in the materials we study, such as gadolinium and cerium. A similar study of such dense materials can be challenging with electron microscopy.
The HXN is revolutionary; there is nothing else out there like it. Because it has a beam spot of just 10 nanometers, what we can do with it is quite pronounced. It represents a whole new class of x-ray microscopy.
Who else is involved in this study?
I feel very fortunate to have worked with and advise many excellent graduate and undergraduate students, post-docs, and research scientists in my lab. We work closely with Kyle S. Brinkman (Clemson University), Fanglin Chen (University of South Carolina), and their lab members, who synthesized and performed other characterizations of the MIEC material used in this study. Initial synchrotron-based transmission x-ray microscopy imaging of this material was performed with Karen Chen-Wiegart and Jun Wang at Brookhaven's former light source, the NSLS. Electron microscopy was performed in collaboration with Dong Su at the Brookhaven's Center for Functional Nanomaterials. This work was supported by the HeteroFoaM Center, an Energy Frontier Research Center (EFRC) directed by Kenneth L. Reifsnider, and funded by the U.S. Department of Energy, Office of Science, Office of Basic Energy Sciences (Award no. DE-SC0001061).
Editor’s Note: NSLS-II and APS are DOE Office of Science User Facilities.
2016-6390 | INT/EXT | Newsroom