The Second Quantum Revolution
The first quantum revolution nearly a century ago led to the 20th-century technological revolution, which was based on the transistor, laser, and atomic clock. These inventions gave us computers, optical fiber communication, and the global positioning system, all of which are vital to the world economy. However, we have come to understand that these technologies do not harness the full power of quantum mechanics.
We are now in the early stages of a second quantum revolution in which we are beginning to be able to employ this power for useful tasks. We do not yet know all of the applications that the second quantum revolution will bring, but we anticipate they could be as revolutionary as the first ones. For example, if we can build practical large-scale quantum computers, we could design new catalysts to efficiently synthesize chemicals such as fertilizers and new drug molecules that bind to specific targets. We could also rapidly solve complex optimization problems of great commercial importance—including logistics, scheduling, traffic congestion, fraud detection, and stock portfolio management—as well as fundamental science questions in nuclear physics, high-energy physics, and other fields.
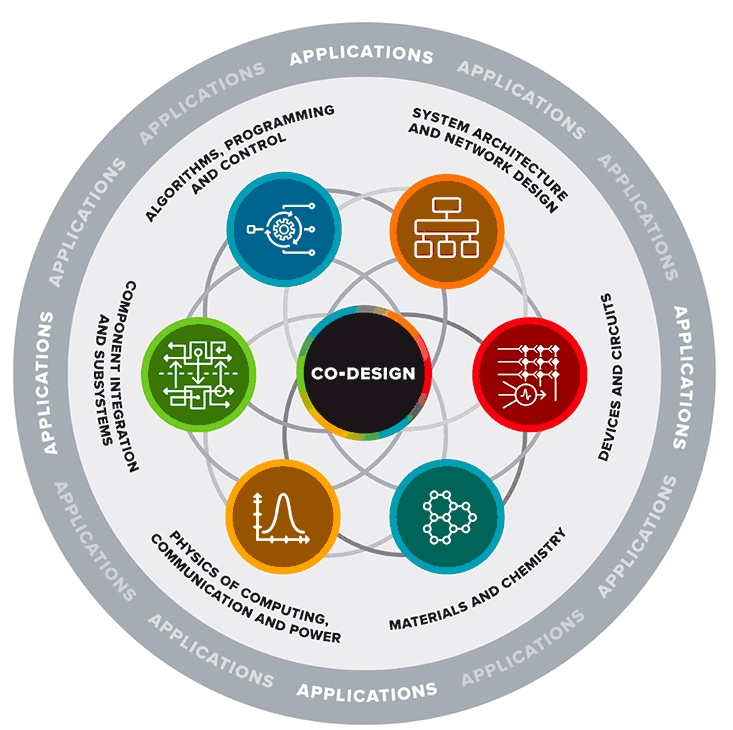
Co-design
Software and Algorithms
Because they work in a fundamentally different way than ordinary (“classical”) computers, quantum computers require an entirely new software architecture. We are developing applications, algorithms, and software technologies that take advantage of the latest hardware advances to harness the tremendous processing power promised by quantum computers. One of our major goals is to perform scalable quantum simulations of lattice gauge theories—which describe fundamental phenomena occurring in nature, such as interactions between elementary particles—for the first time.
Devices
The basic hardware elements of quantum computers are information-storing quantum bits, or qubits, the counterparts to the bits of today’s computers. Applying insights from our materials research thrust, we are fabricating, characterizing, and optimizing devices to process and transmit quantum information for distributed computing. We are focusing on devices that leverage superconductivity—the phenomenon where electricity moves through a material without resistance—and nanophotonics, the control of light at the nanoscale (billionths of a meter). Our goal is to improve various performance parameters, including the amount of time before errors overwhelm qubits and the distance over which quantum systems can communicate.
Materials
Qubits are highly prone to errors as a consequence of interactions with their environment and defects in the qubit materials—atoms, ions, photons, or electrons—themselves. We are uncovering the microscopic mechanisms behind these errors through a feedback loop involving theory, materials synthesis and characterization, and quantum device measurements. Our approach is accelerating the improvement and discovery of materials to enable more stable, reliable, and large-scale quantum computing.