Local Strong Parity Violation and New Perspectives In the Experimental Study of Non-Perturbative QCD
January 21, 2010
By L. Evan Finch, Dmitri E. Kharzeev, Jack Sandweiss, and Sergei A. Voloshin
Parity violation means that our world is distinguishable from its mirror image. The physics world was astonished some 50 years ago by the discovery that the weak interaction (responsible for the beta decay of nuclei as well as for some elementary particle decays) violates parity symmetry. Almost as shocking was the subsequent finding that the CP symmetry, in which a parity transformation is combined with the replacement in the mirror world of all particles by their corresponding antiparticles, is also not respected by the weak interaction. These fundamental discoveries were marked by three Nobel prizes. Strong interactions (that hold the nucleons bound in nuclei and the quarks bound in the nucleons) are found experimentally to a very high precision to obey parity and CP invariances - the best limit on the amount of P and CP violation is currently set by the measurement of the neutron dipole moment. However, in Quantum Chromo-Dynamics (QCD) - the modern theory of strong interactions - P and CP conservation is not natural, and large local violations of these symmetries are possible. It has been predicted that in heavy ion collisions these local violations of P and CP symmetries can occur, and would induce the separation of electric charge along the angular momentum vector of the collision, in effect giving an electric dipole moment to the produced hot gluon matter [1].
Recently, the STAR Collaboration has published very intriguing results [2] on charge dependent azimuthal correlations, which are in qualitative agreement with theoretical expectations from the picture of electric charge separation along the angular momentum vector. Such a separation would be a manifestation of the local strong parity violation. The STAR results, which at present have not been explained by any other known model, may be considered as a first evidence for such physics.
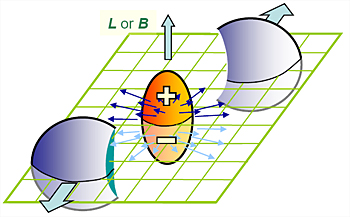
Figure 1: Schematic view of the charge separation along the system's orbital angular momentum. The orientation of the charge separation fluctuates in accord with the sign of the topological charge.
The charge separation stems from the interplay of the strong magnetic field in the early stage of the heavy ion collision and the presence of topological configurations in hot matter ("the chiral magnetic effect" [1,3,4]). This effect is enhanced in the "deconfined" quark-gluon plasma phase of strongly interacting matter in which the separation of quarks over a large (i.e. comparable to the system's size) distance becomes possible.
An important difference of the observed effect from the violation of parity in weak interactions is that in strong interactions Nature makes a choice for either left- or right- handed violation with equal probability in each collision event. Making an analogy with a wind vane: in weak interactions the vane is always pointing in the same direction. In strong interactions, the vane is rotating rapidly - while it does always point in some direction at any given moment, there is no preferred direction on the average. This makes it necessary to study local parity violation event by event and to focus on the fluctuations in parity-odd quantities. Experimentally, such fluctuations can be addressed by the measurement of three-particle correlations [5]. The STAR collaboration used the observable 〈cos(φα+φβ−2ΨRP) 〉, where φα,β are azimuthal angles of two (same or opposite) charged particles, and ΨRP is the reaction plane angle (the azimuthal angle of the plane containing the beam momenta and the vector joining the centers of the two nuclei). This correlator represents the difference between the in-plane and out-of-plane correlations and by construction is not sensitive to non-reaction-plane dependent correlations which otherwise would dominate the measurement. Figure 2, taken from the STAR publication [2] shows a significant difference between the azimuthal dependence of the same-charge (red stars) and opposite-charge (blue stars) pairs; this is consistent with the picture of charge separation relative to the reaction plane, and thus with the local P and CP violation. However, because it is a measurement of fluctuations, a contribution from a mundane parity-even effect is in principle possible. No known effects that could imitate the observed signal have been found to date, but such studies have to be continued.
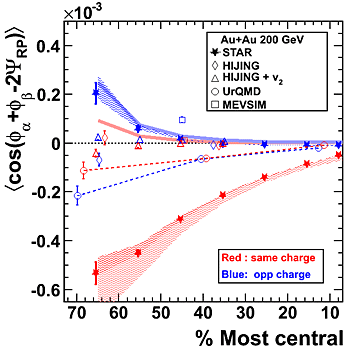
Figure 2: 〈 cos(φα+φβ−2ΨRP) 〉 calculated by STAR for same-charge (red stars) and opposite-charge (blue stars) correlations in 200 GeV Au+Au events as a function of collision centrality (with "head-on" collisions to the far right on the x-axis). Other symbols represent calculations with various theoretical models which do not include local parity violation effects, none of which to date give a qualitative description of the observations.
The STAR results can be considered as only a beginning of a dedicated experimental program aimed first at confirming (or disproving) the interpretation of these results as originating from local parity violation, but also more generally aimed at understanding the role of the non-trivial topological structure of QCD. The RHIC beam energy scan will be an important part of such a program - indeed, at a sufficiently low energy when the deconfined matter can no longer be produced, one expects a suppression of the charge separation effect. Identified hadron and multi-particle correlations studies will also become possible with larger statistics. The charge separation dependence on the magnetic field of the collision system can be tested in collisions of isobaric beams, such as 9644Ru and 9640Zr, which have been performed at GSI and are also possible at RHIC. Another direction that can be envisioned is the study of topological effects in "usual" multi-particle production processes, such as in proton-proton collisions. In particular, one may think of the typical inelastic event as of a sequence of "sphaleron"-dominated "explosions" ordered in rapidity [6,7] (a sphaleron is a particular gluon field configuration which leads to a discrete change in the topology of the QCD ground state). The double diffractive production processes, the study of which is proposed as a major component of the second phase of the pp2pp experiment (now part of STAR) would create a very clean environment for the study of sphaleron-like clusters - indeed, in these processes such clusters can be produced one at a time. The mass of a sphaleron-like cluster is set by its typical size and is about 3 GeV, well accessible in the proposed settings. In these processes one can analyze the multiplicity distributions, test the "flavor democracy" (the production of equal number of qq pairs of all flavors) that is expected in the decay of the sphaleron clusters, and determine whether the angular distributions are isotropic as expected. HBT analysis could be also very interesting, as the sphalerons/instantons are semi-classical fields in nature, and as such are described by coherent gluonic fields.
If established decisively, the local parity violation would constitute the first direct observation of a topological effect in non-Abelian gauge theories. This observation would have an effect reaching far beyond the physics of RHIC - it can modify our view of the Early Universe by providing e.g. a possible primordial source for the magnetic helicity. In addition, it would improve understanding of the origin of baryon asymmetry which is closely analogous to the chirality asymmetry possibly observed at RHIC.
References
- [1]
- D. Kharzeev, Phys. Lett. B 633, 260 (2006) [arXiv:hep-ph/0406125].
- [2]
- B. I. Abelev [STAR Collaboration], arXiv:0909.1717 [nucl-ex]; arXiv:0909.1739 [nucl-ex].
- [3]
- D. Kharzeev and A. Zhitnitsky, Nucl. Phys. A 797, 67 (2007) [arXiv:0706.1026 [hep-ph]].
- [4]
-
D. E. Kharzeev, L. D. McLerran and H. J. Warringa,
Nucl. Phys. A 803, 227 (2008);
K. Fukushima, D. E. Kharzeev and H. J. Warringa, Phys. Rev. D 78, 074033 (2008). - [5]
- S. A. Voloshin, Phys. Rev. C 70, 057901 (2004) [arXiv:hep-ph/0406311].
- [6]
- D. E. Kharzeev, Y. V. Kovchegov and E. Levin, Nucl. Phys. A 690, 621 (2001) [arXiv:hep-ph/0007182].
- [7]
- M. A. Nowak, E. V. Shuryak and I. Zahed, Phys. Rev. D 64, 034008 (2001) [arXiv:hep-ph/0012232].
2010-1588 | INT/EXT | Newsroom