- About Us
- Our History
-
Overview
Our origins as the first large-scale research facility in the north-eastern United States.Discoveries
Brookhaven is home to discoveries that led to seven Nobel Prizes.Unique Facilities
The Lab was conceived to design, construct and operate large scientific research machines.
-
- Awards
- Leadership Team
- Brookhaven Science Associates
- Stakeholder Relations
- Values & Conduct

A History of Leadership in Particle Accelerator Design
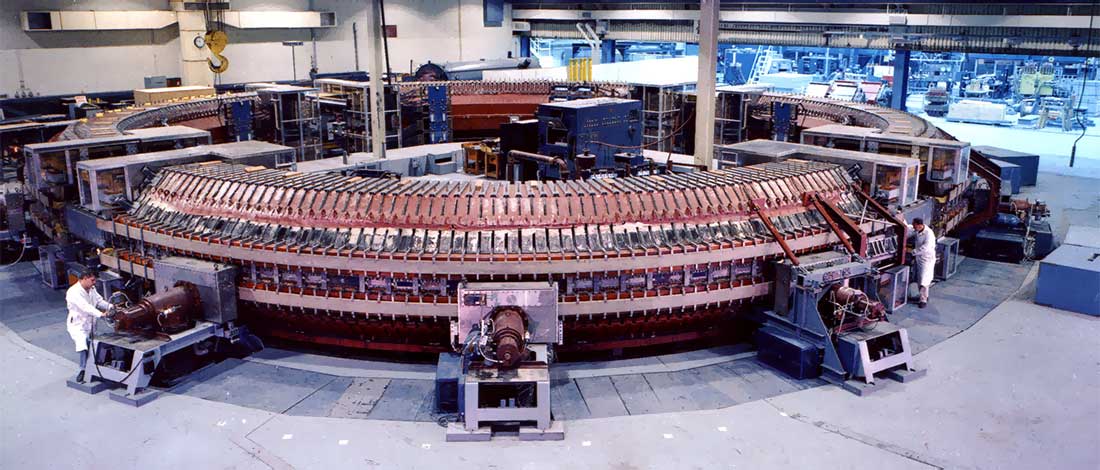
Cosmotron (1952-1966)
Early in Brookhaven Lab history, the consortium of universities responsible for founding the new research center, decided that Brookhaven should provide leading facilities for high energy physics research. In April 1948, the Atomic Energy Commission approved a plan for a proton synchrotron to be built at Brookhaven. The new machine would accelerate protons to previously unheard of energies—comparable to the cosmic rays showering the earth's outer atmosphere. It would be called the Cosmotron.
The Cosmotron was the first accelerator in the world to send particles to energies in the billion electron volt, or GeV, region. The machine reached its full design energy of 3.3 GeV in 1953.
Not only was the Cosmotron the world's highest energy accelerator, it was also the first synchrotron to provide an external beam of particles for experimentation outside the accelerator itself.
The Cosmotron was the first machine to produce all the types of negative and positive mesons known to exist in cosmic rays, making possible the discoveries of the K0L meson and the first vector meson. It was also the first accelerator to produce heavy unstable particles, some of which were formerly called "V" particles, and this led directly to the experimental confirmation of the theory of associated production of strange particles.
After 14 years of service to the physics community, the Cosmotron ceased operation in 1966 and was dismantled in 1969. Knowledge gained from the Cosmotron's experiments would lead to revolutionary design improvements and pave the way for construction of Brookhaven's next big accelerator: the Alternating Gradient Synchrotron.
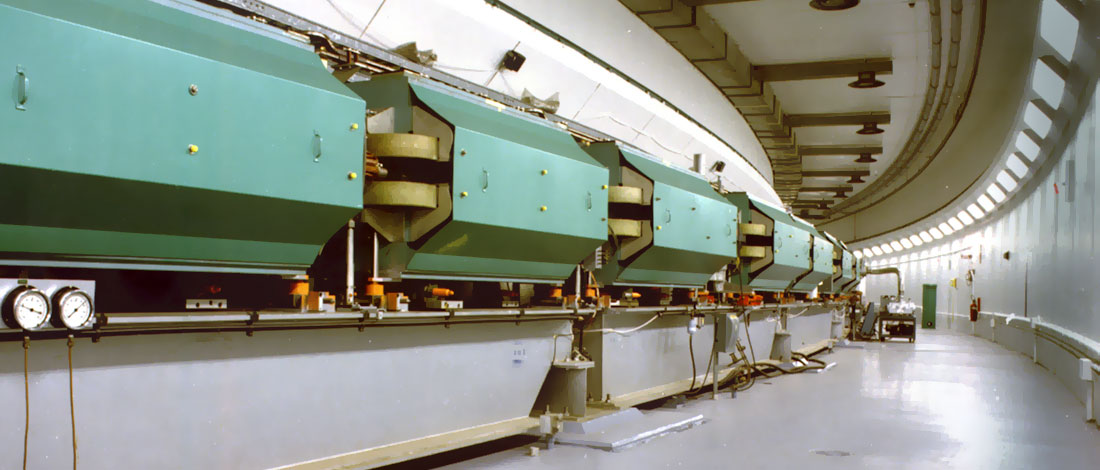
Alternating Gradient Synchrotron (1960-present)
The Alternating Gradient Synchrotron (AGS) was built on the innovative concept of the alternating gradient, or strong-focusing, principle, developed by Brookhaven physicists Ernst Courant, M. Stanley Livingston, and Hartland Snyder in the 1950s. This breakthrough concept in accelerator design allowed scientists to accelerate protons to energies that would have been otherwise unachievable. The AGS became the world's premiere accelerator when it reached its design energy of 33 billion electron volts (GeV) in July of 1960. AGS continues to serve an important role in the accelerator complex that sends particles to the Relativistic Heavy Ion Collider (RHIC).
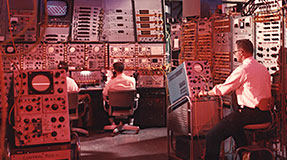
AGS control room, circa 1966.
Until 1968, the AGS was the highest energy accelerator in the world, slightly higher than its 28 GeV sister machine, the Proton Synchroton at CERN, the European Organization for Nuclear Research. AGS dscoveries earned researchers three Nobel Prizes and today still serves as the injector for Brookhaven's Relativistic Heavy Ion Collider. It also remains the world's highest intensity high-energy proton accelerator. The AGS and its accompanying Booster accelerator are the only U.S. heavy ion accelerators suitable for simulating the biological effects of space radiation.
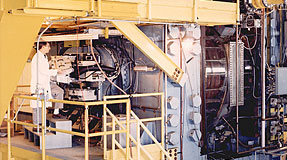
80-inch Bubble Chamber
One of the most famous early detectors used at the AGS to study sub-atomic phenomena was the 80-inch bubble chamber. When its first photograph of particle interactions was taken in June 1963, the 80-inch bubble chamber was the largest such detector in the world. In 1964,a team of researchers led by future Laboratory director Nicholas Samios used the 80-inch to discover the omega-minus particle. This finding supported the first attempt by physicists to organize the increasingly long list of subatomic particles into an orderly pattern, similar to that used to arrange elements in the periodic table.
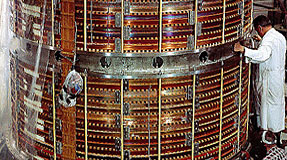
7-foot Bubble Chamber
Later, another detector, the 7-foot bubble chamber, began routine operations in 1974. The following year, the 7-foot chamber was used to discover the charmed baryon, a particle composed of three quarks, one of which was the "charmed" quark. This result helped physicists confirm that new member of the quark family.
Three discoveries made at the AGS—the muon-neutrino, a symmetry-breaking called CP violation and the J/psi particle—each won the Nobel Prize in physics.
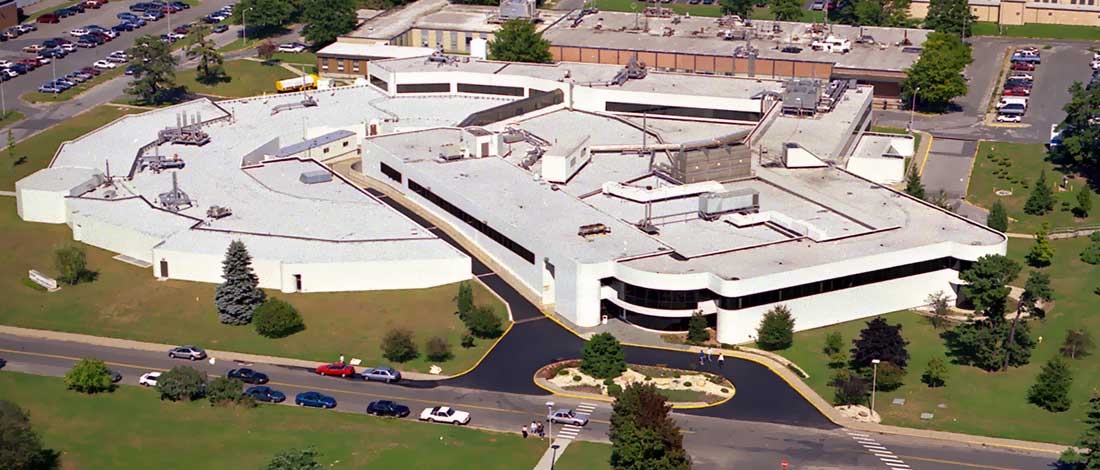
National Synchrotron Light Source (1982-2014)
One of the world’s most widely used scientific research facilities, the National Synchrotron Light Source (NSLS) hosted about 2,400 researchers annually from more than 400 universities, laboratories, and companies. Beginning in the 1980s, research conducted at NSLS yielded advances in biology, physics, chemistry, geophysics, medicine, and materials science.
An accelerator takes stationary charged particles, such as electrons, and drives them to velocities near the speed of light. Forced by magnets to travel around a circular storage ring, the charged particles give off electromagnetic radiation and lose energy. This energy is emitted in the form of light—a phenomenon known as synchrotron radiation.
While it cannot be seen by the human eye, when used in certain ways and viewed by special detectors, this light reveals structures and features of individual atoms, molecules, crystals, cells and more—especially when the wavelength and corresponding energy of the light are matched to the size of the sample being viewed. Because synchrotron light is very intense and well focused, it is preferred to light produced by conventional laboratory sources.
When the U.S. Department of Energy recognized the need for "second generation" electron synchrotrons dedicated to the production of light, it budgeted construction funding for Brookhaven's National Synchrotron Light Source (NSLS). Ground was broken for NSLS on September 28, 1978, and the vacuum ultraviolet (VUV) ring began operations in late 1982, while the x-ray ring was commissioned in 1984.
Research conducted at NSLS yielded advances in biology, physics, chemistry, geophysics, medicine, and materials science. Examples of research performed at NSLS include investigations into the chemical origins of nerve impulses, studies of the crystal structure of new materials such as high-temperature superconductors, studies of arthritis and osteoporosis, and techniques to make faster, smaller computer chips.
Two Brookhaven scientists, Renate Chasman and G. Kenneth Green were responsible for developing the technology that made the NSLS possible: the "double focusing achromat," more commonly known as the Chasman-Green lattice. The lattice is the periodic arrangement of magnets that bend, focus and correct the electron beam. When special magnets are inserted into the accelerator ring, the electron beam "wiggles" and emits even more intense synchrotron radiation. Chasman and Green's inclusion of these devices in their design of the storage rings enabled the NSLS to deliver world-class beams of light and their innovation is now a standard component of synchrotrons the world over.
More about NSLS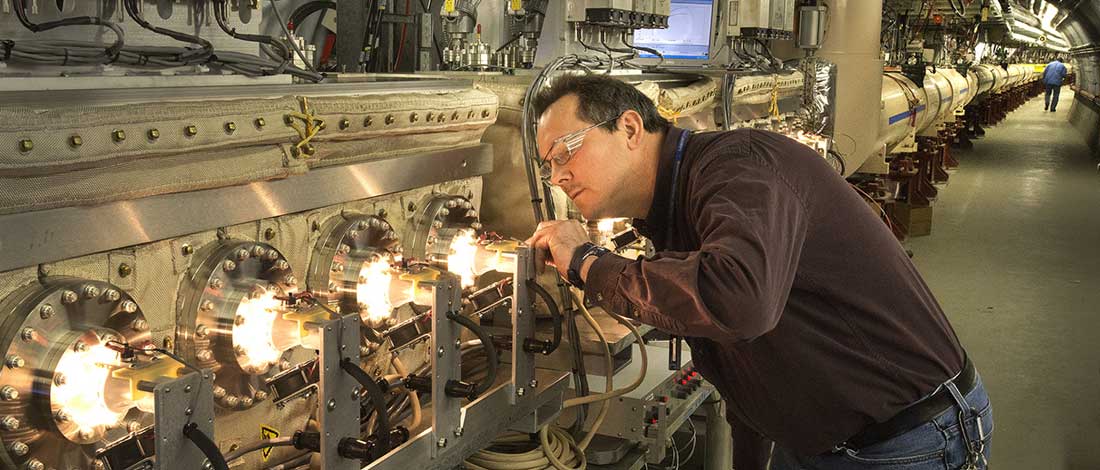
Relativistic Heavy Ion Collider (2000-present)
The Relativistic Heavy Ion Collider (RHIC), which recreates ultra-hot matter that existed at the dawn of time, achieved its first successful operation in the summer of 2000, capping ten years of development. However, the history of RHIC stretches back more than 30 years beginning with an idea for a machine called the Intersecting Storage Accelerator.
Two Beams Are Better Than One
When single ring accelerators like the Alternating Gradient Synchrotron propel protons against a stationary target to investigate nuclear properties, much of the usable energy in the reaction is lost. It was recognized by early accelerator physicists that much higher reaction energies could be achieved by colliding two accelerated beams head-on.
The idea of using storage rings for a colliding beam accelerator was considered at a summer study held at Brookhaven in 1963. The idea was revived in 1970 leading to a recommendation that Brookhaven apply its pioneering development work in superconducting magnets to build two proton intersecting storage rings. This was the beginning of the ISABELLE project.
The ISABELLE Project
In 1974, the U.S. High Energy Physics Advisory Panel (HEPAP) recommended that ISABELLE be built at Brookhaven. The following year, the first full-size superconducting magnet was successfully tested and the magnetic fields exceeded the expected design strength. In 1977 the third HEPAP panel recommended that ISABELLE be given top priority. Groundbreaking for ISABELLE was held in October 1978. In 1981, however, technical problems were encountered in the fabrication of the superconducting magnets needed to power the machine, bringing ISABELLE to a halt. HEPAP then shifted its focus to the Superconducting Supercollider, which would itself later be canceled.
A Concept Reborn
Physicists at Brookhaven persevered, continuing to push for an advanced accelerator design. In 1984, the first proposal was submitted for the machine now known as RHIC. RHIC's main mission was to search for a state of matter called quark-gluon plasma, believed to have existed just moments after the Big Bang. It was considered very cost-effective to build RHIC at Brookhaven because of the existing accelerator infrastructure which could be used to inject protons and heavy ions into the machine as well as the fact that a tunnel (originally excavated for ISABELLE) was already completed. Brookhaven received funding to proceed with the construction of RHIC in 1991 and physics operations began in 2000.