Physicists Receive Patent for Improved Cancer Therapy Device
New design would deliver more precise radiation doses at lower cost
November 24, 2008
UPTON, NY — Four physicists at the U.S. Department of Energy’s (DOE) Brookhaven National Laboratory have been awarded U.S. Patent No. 7,432,516 B2 for the design of a “medical synchrotron” capable of delivering precision doses of proton radiation to cancerous tumors with minimal damage to surrounding healthy tissue. The new device would be more precise and less costly than existing proton-therapy systems, potentially increasing the availability and benefits of this treatment for cancer patients worldwide. The Brookhaven scientists are now seeking industrial partners to license and commercialize the technology.
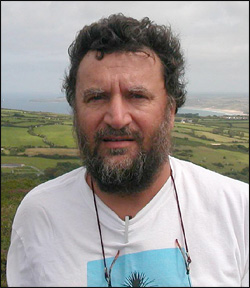
Stephen Peggs
“In the realm of cancer treatment, proton therapy is considered ‘surgery without a knife’ because proton beams can deliver cell-killing energy with extreme precision, unlike conventional x-ray radiation therapy,” said Brookhaven physicist Stephen Peggs, one of the lead scientists on the project. Peggs, while working at DOE’s Fermi National Accelerator Laboratory, witnessed the completion of the nation’s first hospital-based proton-therapy synchrotron, installed at California’s Loma Linda University Medical Center in 1990.
“Almost as soon as the Loma Linda synchrotron went out the door, we started thinking about ways to build a better machine,” Peggs said. The current design — developed and refined as Peggs and other physicists worked on large-scale accelerators for physics experiments, including the Relativistic Heavy Ion Collider (RHIC) at Brookhaven Lab — is the culmination of that effort.
“Our new design has improvements in beam-focusing technology to make the smallest possible beam size — that is, the sharpest possible ‘knife,’” said Peggs. Because smaller beams deliver radiation with increased precision, this improvement could have a significant impact by shortening the duration of treatment, increasing its effectiveness, or both. The new design also promises to be less costly and more reliable, which should increase its availability.
How it works
The idea behind radiation therapy is to deliver a lethal dose of radiation to cancerous cells. In conventional x-ray radiation therapy, many healthy surrounding cells are also exposed to the radiation because x-ray beams deposit their energy as they travel through tissue. In fact, most of the dose of x-rays is deposited near the surface of the body. Though cancerous cells tend to be more susceptible to the damaging effects of radiation (or less able to repair it), the collateral damage to healthy tissues limits the dose physicians can use to destroy the tumor.
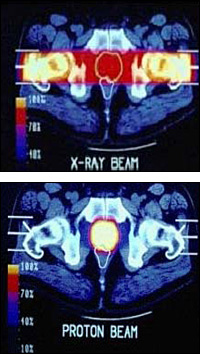
The comparison of energy deposited at a tumor from proton therapy (bottom) and an x-ray beam radiation (top), with yellow indicating the highest dose. (Image courtesy of the National Association for Proton Therapy.)
Proton therapy offers an advance over conventional x-rays because proton beams deposit most of their energy where the beam stops. The original proton therapy synchrotrons were designed to deliver cell-killing doses of radiation to tumors in three dimensions by aiming proton beams from multiple directions to stop at the depth of the tumor tissue. That precision targeting allows doctors to deliver higher doses to the tumor cells while sparing healthy surrounding tissue.
But accelerators are often costly to build and difficult to maintain, explaining why the design principles for hospital-based accelerators must be radically modified, and why relatively few hospitals have them.The new accelerator design developed by the Brookhaven team offers two main advantages: “rapid cycling” and “strong focusing.”
Rapid cycling allows proton beams to be injected and extracted from the synchrotron in just one turn around the circular particle accelerator. Unlike the earlier machines, which required multiple turns, this eliminates the need for sensitive feedback systems to control the beam currents.
“This makes the machine more robust and reliable to operate. It’s more of a turn-key operation,” Peggs said. “Turn it on and it consistently starts up like a transformer, rather than booting up like a PC.”
Strong focusing refers to the ability to shape the proton beam and keep it focused to pinpoint dimensions. In contrast to the Loma Linda machine, where beams measure up to a centimeter across, the new design can achieve beams as narrow as one millimeter.
Pinpoint accuracy reduces collateral damage and allows physicians more flexibility in the doses they use. Higher doses could yield more effective therapy, possibly in fewer treatments.
Compact beam size has other benefits as well: smaller components (beam pipes, magnets, etc.) for the whole device. That makes everything lighter, and less expensive, Peggs said. Smaller size will also eliminate the need for water-cooling most magnets; air-cooling will be sufficient. That adds up to even more cost savings.
“Our biggest challenge now is to find partners in an industrial consortium to help us build one of these new machines,” said Peggs. “It’s part of our mission as a national laboratory to put together teams and serve national needs in terms of technology transfer. We would help to build the first specialized, high-tech items, but most of an accelerator is made from conventional technology, and that can be done by industry. So we are looking for a consortium to see this move into the commercial sector — and hospitals across the country,” he said.
In addition to Peggs, Michael Brennan, Joseph Tuozzolo, and Alexander Zaltsman, all in Brookhaven Lab’s Collider-Accelerator Department, collaborated on the project. Their ideas and designs stem directly from their experience working on particle accelerators for physics experiments.
“It’s the fact that we do the science for accelerators like RHIC that allows us to do this for medical applications,” Peggs said.
The physicists’ work at RHIC and on the development of the improved medical synchrotron is supported by the DOE’s Office of Science (Office of Nuclear Physics).
For licensing information about this technology, please contact Lori-Anne Neiger, lneiger@bnl.gov, 631 344-3035.
2008-10870 | INT/EXT | Newsroom