2014's Top-10 Scientific Achievements at Brookhaven Lab
January 5, 2015
UPTON, NY—From new insights into the building blocks of matter to advances in understanding batteries, superconductors, and a protein that could help fight cancer, 2014 was a year of stunning successes for the U.S. Department of Energy's Brookhaven National Laboratory. Oh, and did we mention the opening of a brand new facility that will push the limits of discovery across the scientific spectrum? For details on these and the rest of our Top-10 breakthroughs, check out the items below. You can follow Brookhaven Lab on Facebook, Twitter, and Tumblr to learn about the discoveries of 2015 as they happen.
1. National Synchrotron Light Source II Achieves 'First Light'
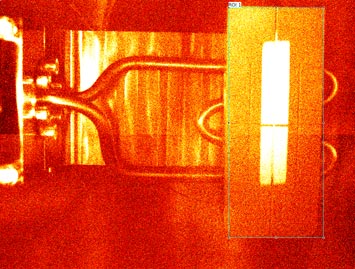
Inside the beamline enclosure, a phosphor detector (the rectangle at right) captured the first x-rays (in white) which hit the mark dead center.
Opening a new era of scientific discovery at Brookhaven Lab, the brightest synchrotron light source in the world delivered its first x-ray beams on October 23, 2014. Soon researchers from around the world will start using the powerful x-rays produced by the National Synchrotron Light Source II (NSLS-II) to advance their research on everything from new energy storage materials to developing new drugs to fight disease. As of this writing, all seven of the initial experimental stations designed to receive x-rays from the ½-mile-circumference electron storage ring had received 'first light.' Scientists running these "beamlines" will begin experiments this year. An additional 23 beamlines are under construction or in the planning stages, and there's room at NSLS-II for 30 more. With the photons shining, 2015 promises to be a bright year for science at NSLS-II.
2. Physicists Narrow Search for Solution to Proton Spin Puzzle
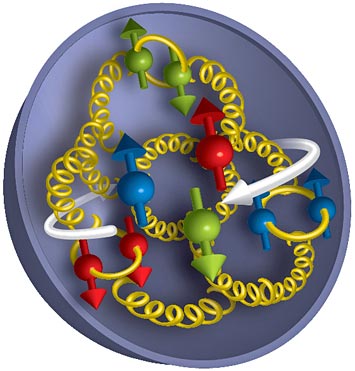
How the spins of the building blocks of matter add up: Measurements from RHIC's STAR and PHENIX experiments reveal that gluons (yellow corkscrews) contribute about as much as quarks (red, green, and blue) to the overall spin of a proton. But there is still a mystery to explain what accounts for the rest of the "missing" spin.
Results from experiments at the Relativistic Heavy Ion Collider (RHIC), a 2.4-mile-circumference particle collider at Brookhaven Lab, reveal new insights about how quarks and gluons—the subatomic building blocks of protons—contribute to proton "spin." The new precision measurements will help solve a mystery that has puzzled physicists since the 1980s, showing for the first time that gluons make a significant contribution to proton spin and that transient "sea quarks"—which form primarily when gluons split—also play a role. Pinpointing where spin comes from could yield new information about the mechanisms of the complex subatomic particle interactions within protons, the effects of spin on other properties, and perhaps even ways to control those properties for future, unforeseen applications. RHIC is the world's only facility capable of colliding spin-polarized protons to perform these precision studies.
3. New Method Provides Nanoscale Details of Electrochemical Reactions in Electric Vehicle Battery Materials
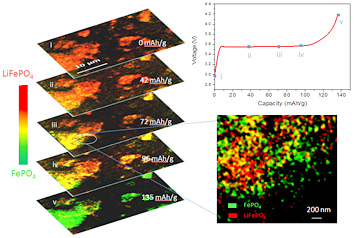
In operando 2D chemical mapping of multi particle lithium iron phosphate cathode during fast charging (top to bottom). The called-out close-up frame shows that as the sample charges, some regions become completely delithiated (green) while others remain completely lithiated (red). This inhomogeneity results in a lower overall battery capacity than can be attained with slower charging, where delithiation occurs more evenly throughout the electrode.
Using a new method to track the electrochemical reactions that take place in a common electric vehicle battery material, scientists at Brookhaven have gained new insight into why fast charging inhibits this material's performance. The team used a combination of techniques to study the material under operating conditions and at the nanometer scale at the National Synchrotron Light Source (NSLS), Brookhaven's original synchrotron x-ray light source, which closed at the end of September after 32 years of successful operation. The resulting high-resolution images and electrochemical "fingerprint" show, pixel by pixel, where lithium ions remain in the material, where they've been removed, and other potentially interesting electrochemical details. The findings provide the first direct experimental evidence to support a particular model of the electrochemical reaction, and could inform battery makers' efforts to optimize materials for faster-charging batteries with higher capacity.
4. Tracking the Transition of Early-Universe Quark Soup to Matter-as-we-know-it
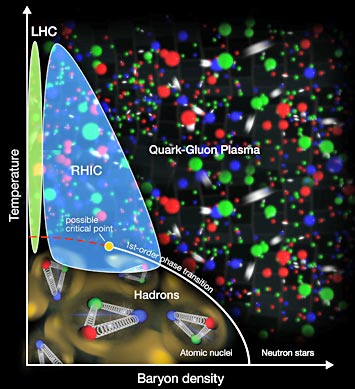
The STAR collaboration's exploration of the "nuclear phase diagram" shows signs of a sharp border—a first-order phase transition—between the hadrons that make up ordinary atomic nuclei and the quark-gluon plasma (QGP) of the early universe when the QGP is produced at relatively low energies/temperatures. The data may also suggest a possible critical point, where the type of transition changes from the abrupt, first-order kind to a continuous crossover at higher energies.
To figure out how the hot soup of subatomic particles that filled the early universe transformed into the ordinary matter of today's world, nuclear physicists run the process in reverse at the Relativistic Heavy Ion Collider (RHIC) at Brookhaven Lab. They accelerate ordinary atomic nuclei to close to the speed of light, and smash these ions together to recreate matter at the extreme temperatures and densities that existed just after the Big Bang. In 2014, new analyses of the particles that emerge from the trillion-degree collision zone turned up interesting details about how the transition from primordial matter to atoms takes place. In particular, the RHIC STAR collaboration's analysis over a very wide range of collision energies that can be created at this versatile facility suggests that the type of transition changes depending on the energy the particles have when they collide. Future experiments making use of recently installed detector components should help the physicists hone in on a "critical point" where that change of transition type takes place.
5. Researchers Pump Up Oil Accumulation in Plant Leaves
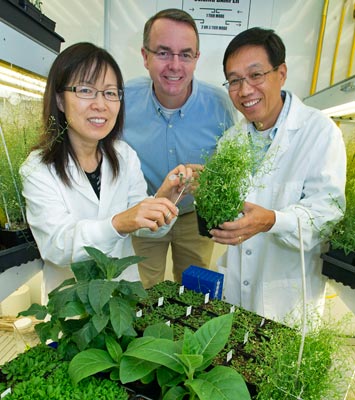
Brookhaven researchers Jilian Fan, John Shanklin, and Changcheng Xu have developed a method for getting experimental plants to accumulate more leaf oil. Their strategy could have a significant impact on the production of biofuels.
Detailed genetic studies at Brookhaven last year revealed previously unknown biochemical details about how plant leaves make and break down oils—including new ways to increase the accumulation of oil in the leaves. Increasing the oil content of leaves, plants' most abundant source of biomass, could help fulfill the nation's increasing demand for renewable energy feedstocks. Using new methods revealed by the study, the scientists grew experimental plants whose leaves accumulated 9 percent oil by dry weight—an approximately 150-fold increase in oil content compared to wild type leaves. If the scientists can replicate these results in crop plants, whose growth time is longer, they may be able to achieve oil accumulation as high as 40 percent by weight. That would be a boon for the production of biofuels, because energy-dense oils give more "bang per bushel" than less-energy-dense leaf carbohydrates.
6. Scientists Find Solution to Two Long-Standing Mysteries of Cuprate High-Temperature Superconductivity
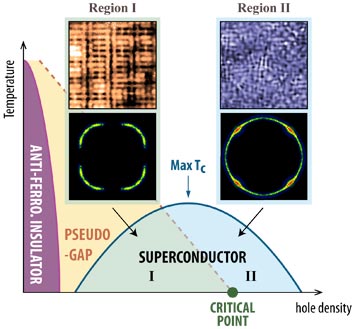
Scientists used a precision microscope to simultaneously explore electrons' arrangements and movements as charge carriers called holes were added to transform a copper-oxide material from an insulator to a superconductor.
A team led by scientists at Brookhaven Lab and Cornell University trying to unravel the intricacies of high-temperature superconductivity—the ability of certain materials to carry electrical current with no energy loss—has been particularly puzzled by a mysterious phase that appears to compete with superconductivity. Now, using precision techniques they've revealed detailed characteristics of the electrons in one of these materials as it transforms from an insulator through the mysterious "pseudogap" phase and eventually into a full-blown superconductor. The results link two distinct personality changes in the material's electrons—the disappearance of an exotic periodic arrangement of certain electrons within the pseudogap phase (sometimes referred to as "stripes"), and the sudden ability of all the material's electrons to move freely in any direction. The finding could be a significant step toward unlocking the potential of these materials for energy-saving applications.
7. Physicists Detect Process Even Rarer than the Long-Sought Higgs Particle
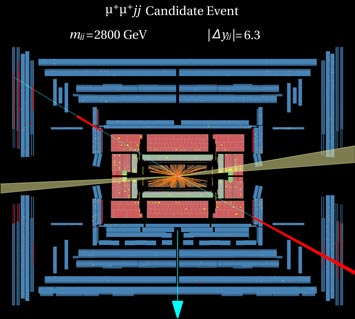
Candidate event for WW → WW scattering in the ATLAS detector at the Large Hadron Collider. (Image: ATLAS Experiment © 2014 CERN)
Scientists running the ATLAS experiment at the Large Hadron Collider (LHC), the world's largest and most powerful "atom smasher," including a large contingent from Brookhaven Lab, report the first evidence of a process that can be used to test the mechanism by which the recently discovered Higgs particle imparts mass to other fundamental particles. More rare than the production of the Higgs itself, this process—a scattering of two same-charged particles called W bosons off one another—also provides a new stringent test of the Standard Model of particle physics. Brookhaven physicists involved in the analysis say the results so far are in good agreement with what's predicted by the Standard Model and its description of how the Higgs works. But the team is looking forward to more detailed studies using the vastly expanded datasets that will be generated when the LHC begins operating at significantly higher energies in the spring of 2015.
8. Scientists Pinpoint the Creeping Nanocrystals Behind Lithium-Ion Battery Degradation
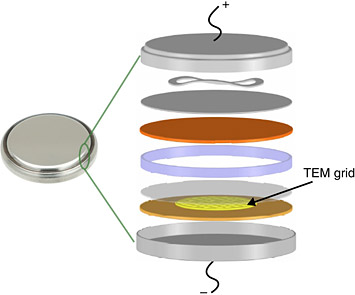
In the experimental coin cell setup, a carbon supported transmission electron microscopy (TEM) grid loaded with a small amount of the nickel-oxide material was pressed against the bulk anode and submerged in the same electrolyte environment.
To stop or slow the steady degradation of the lithium-ion batteries that power our portable electronics, scientists must track and tweak their imperfect chemistry with nanoscale precision. Recent studies to map these crucial billionths-of-a-meter dynamics—conducted in part using world-leading electron microscopy techniques at Brookhaven's Center for Functional Nanomaterials—revealed surprising and never-before-seen evolution and degradation patterns. Contrary to large-scale observation, the nanoscale studies reveal that the lithium-ion reactions erode the materials non-uniformly, seizing upon intrinsic vulnerabilities in atomic structure in the same way that rust creeps unevenly across stainless steel. Armed with a precise map of the materials' erosion, the scientists can plan new ways to break the patterns and improve performance.
9. Scientists Capture Ultrafast Snapshots of Light-driven Superconductivity
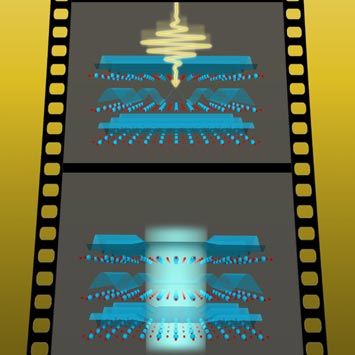
When a mid-infrared laser pulse strikes the material (top), it "melts" these conflicting ripples and induces superconductivity (bottom).
In another step toward improved understanding of high-temperature superconductors, a team led by scientists from the Max Planck Institute for the Structure and Dynamics of Matter in Germany, SLAC National Accelerator Laboratory, and Brookhaven used carefully timed laser pulses at SLAC's Linac Coherent Light Source (LCLS) to take x-ray snapshots of the atomic and electronic structure inside a promising copper-oxide material just as superconductivity emerged. They discovered that so-called "charge stripes" of increased electrical charge melted away as superconductivity appeared. Further, the results help rule out the theory that shifts in the material's atomic lattice hinder the onset of superconductivity. Armed with this new understanding, scientists may be able to develop new techniques to eliminate charge stripes and help pave the way for room-temperature superconductivity. The demonstrated ability to rapidly switch between the insulating and superconducting states could also prove useful in advanced electronics and computation.
10. Scientists Reveal Details of Calcium 'Safety-Valve' in Cells
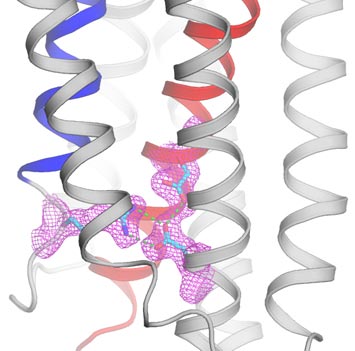
A calcium-leak channel prevents calcium overload in cellular organelles for protection of life. Viewing from within the membrane, the structure is shown as ribbons for the closed-conformation. The di-aspartyl pH-sensor unit and the arginine/aspartate lock are shown as sticks covered by electron densities in magenta.
The accumulation of calcium in cells is a key signaling agent that can trigger programmed cell death, or apoptosis. Understanding what happens when this process goes awry could lead to new ways to control uncontrolled cell growth, a hallmark of cancer. Toward that goal, a team of scientists from Brookhaven Lab, Columbia University, New York University, Baylor College of Medicine, Technical University of Munich, and the New York Structural Biology Center used x-rays at Brookhaven's National Synchrotron Light Source (NSLS) to decipher the structure of a key cellular protein that serves as a molecular safety valve for keeping calcium levels steady. The protein works similar to a pressure safety value used in a standard steam boiler, allowing gradual leakage of calcium and adjusting the flow in response to changes in acidity in the cell. Designing drugs that inhibit this protein would cause calcium levels to rise and promote cell death—a potentially promising strategy for fighting cancers in which such proteins are overexpressed.
These studies and projects were supported primarily by the DOE Office of Science. NSLS, NSLS-II, RHIC, CFN and LCLS are DOE Office of Science User Facilities. See links to full stories for additional information, including other funding sources.
Brookhaven National Laboratory is supported by the Office of Science of the U.S. Department of Energy. The Office of Science is the single largest supporter of basic research in the physical sciences in the United States, and is working to address some of the most pressing challenges of our time. For more information, please visit science.energy.gov.
2015-11687 | INT/EXT | Newsroom