A Recipe for Quantum Scaling
ARQUIN provides a framework for simulating a distributed quantum computing system
October 17, 2024
By Sarah Wong, Pacific Northwest National Laboratory
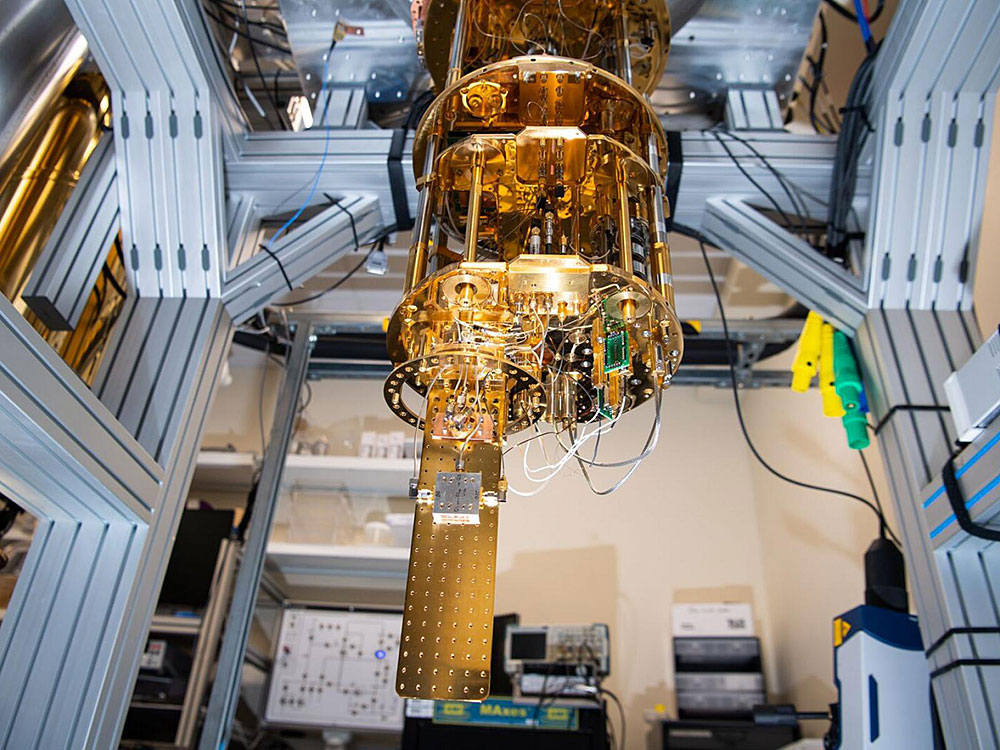
Dilution refrigerators keep superconducting qubits cold. (Photo by Andrea Starr | Pacific Northwest National Laboratory)
Editor’s note: The following press release was originally issued by the U.S. Department of Energy’s (DOE) Pacific Northwest National Laboratory (PNNL). This project was led by Michael DeMarco, who was a postdoctoral researcher at DOE’s Brookhaven National Laboratory when the research was conducted. Brookhaven Lab is the lead institution for the Co-design Center for Quantum Advantage (C2QA), a National Quantum Information Science Research Center funded by the DOE Office of Science. PNNL is a partner institution of C2QA. For more information on Brookhaven’s involvement, contact Danielle Roedel (droedel@bnl.gov).
One of the most difficult problems with quantum computing relates to increasing the size of the quantum computer. Researchers globally are seeking to solve this “challenge of scale.”
To bring quantum scaling closer to reality, researchers from 14 institutions collaborated through the Co-design Center for Quantum Advantage (C2QA), a Department of Energy (DOE), Office of Science, National Quantum Information Science Research Center. Together, they constructed the ARQUIN framework—a pipeline to simulate large-scale distributed quantum computers as different layers. Their results were published in ACM Transactions on Quantum Computing.
Connecting qubits
The research team, led by Michael DeMarco from Brookhaven National Laboratory and the Massachusetts Institute of Technology (MIT), started with a standard computing strategy of combining multiple computing “nodes” into one unified computing framework.
In theory, this multi-node system can be emulated to enhance quantum computers—but there’s a catch. In superconducting quantum systems, qubits must be kept incredibly cold. This is usually done with the help of a cryogenic device called a dilution refrigerator. The problem is that scaling a quantum computing chip to a sufficiently large size within a single fridge is hard.
Even in larger fridges, the superconducting electric circuits within a single chip become difficult to maintain. To create a powerful multi-node quantum computer, researchers need to not only connect nodes inside of one dilution refrigerator, but also to connect the nodes across multiple dilution refrigerators.
Assembling the quantum ingredients
No one institution could carry out the full breadth of research needed for the ARQUIN framework. The ARQUIN team included researchers from Pacific Northwest National Laboratory (PNNL), Brookhaven, MIT, Yale University, Princeton University, Virginia Tech, IBM, and more.
“A lot of quantum research is being done in isolation, with research groups only looking at one piece of the puzzle,” said Samuel Stein, quantum computer scientist at PNNL. “It’s almost like gathering ingredients without knowing how they will work together in a recipe. When experiments are done on only one aspect of the quantum computer, you don’t get to see how the results may impact other parts of the system.”
Instead, the ARQUIN team broke down the problem of constructing a multi-node quantum computer into different “layers,” with each institution working on a different layer based on their area of expertise.
“It’s a huge optimization problem,” said Mark Ritter, chair of the Physical Sciences Council at IBM. “The team had to do an in-depth assessment of the field to look at where we were in terms of technology and algorithms, then do simulations to find out where the bottlenecks were and what could be improved.”
The ARQUIN framework focused on superconducting quantum devices connected by microwave to optical links. Each institution concentrated on a different ingredient of the quantum computing recipe. For example, while some researchers investigated how to optimize microwave-to-optical transduction, others created algorithms that exploit the distributed architecture.
"Such cross-domain systems research is essential to charting roadmaps toward useful quantum information processing applications and is uniquely enabled by the DOE's national quantum initiatives," said Professor Isaac Chuang of MIT.
For their part of the ARQUIN framework, PNNL researchers including Stein, Ang Li, and James (Jim) Ang designed and built the simulation pipeline and generated the Quantum Roofline Model that connected all the ingredients together—essentially creating a framework for trying out different recipes for future quantum computers.
From his unique vantage point, PNNL physicist Chenxu Liu understands the need for multi-institutional collaborations well. He worked on the ARQUIN framework while he was a postdoctoral researcher at Virginia Tech.
“While each research group had expertise in their portion of the project, no one had a very deep understanding of what all of the other groups within the project were doing,” said Liu. “However, each group’s work needed to be embedded into the whole pipeline view of the quantum computer in order to make it meaningful.”
After compiling the different pieces of the project together, ARQUIN became a framework for simulating and benchmarking future multi-node quantum computers. This marks a promising first step toward enabling efficient and scalable quantum communication and computation by integrating modular systems.
Expanding the quantum recipe
Though a functional multi-node quantum computer outlined in the ARQUIN paper has not yet been created, this research provides a road map for future quantum hardware/software co-design.
“Creating a layer-based hierarchical simulation environment—including microwave-to-optical simulation, distillation simulation, and system simulation—was a crucial component in this work,” said Li. “It allowed the ARQUIN team to understand and evaluate the tradeoffs between various design factors and performance metrics regarding the complex distributed quantum computing communication stack.”
Some of the software products created for ARQUIN have already been used by members of the team for other projects. Many of the ARQUIN authors collaborated on another project, called HetArch, to further investigate different superconducting quantum architectures.
“This is an example of applying the principles of co-design from exascale computing to our ARQUIN/HetArch design space explorations,” said Ang.
The ARQUIN study was supported by the Department of Energy, Office of Science, National Quantum Information Science Research Center, Co-design Center for Quantum Advantage (C2QA). HetArch was supported by C2QA and the Advanced Scientific Computing Research, Accelerated Research for Quantum Computing Program. HetArch was also supported in part by the National Science Foundation projects Enabling Practical-Scale Quantum Computation, Software-Tailored Architecture for Quantum Co-design, and the Quantum Leap Challenge Institute for Hybrid Quantum Architectures and Networks.
About PNNL
Pacific Northwest National Laboratory draws on its distinguishing strengths in chemistry, Earth sciences, biology and data science to advance scientific knowledge and address challenges in sustainable energy and national security. Founded in 1965, PNNL is operated by Battelle for the Department of Energy’s Office of Science, which is the single largest supporter of basic research in the physical sciences in the United States. DOE’s Office of Science is working to address some of the most pressing challenges of our time. For more information, visit https://www.energy.gov/science/. For more information on PNNL, visit PNNL's News Center. Follow us on Twitter, Facebook, LinkedIn and Instagram.
2024-22138 | INT/EXT | Newsroom