NSLS-II User Profile: Roopali Kukreja, UC Davis
In the "Leading Lights" series, visiting researchers sit down with NSLS-II staff for a Q&A on their research and user experience
May 18, 2022
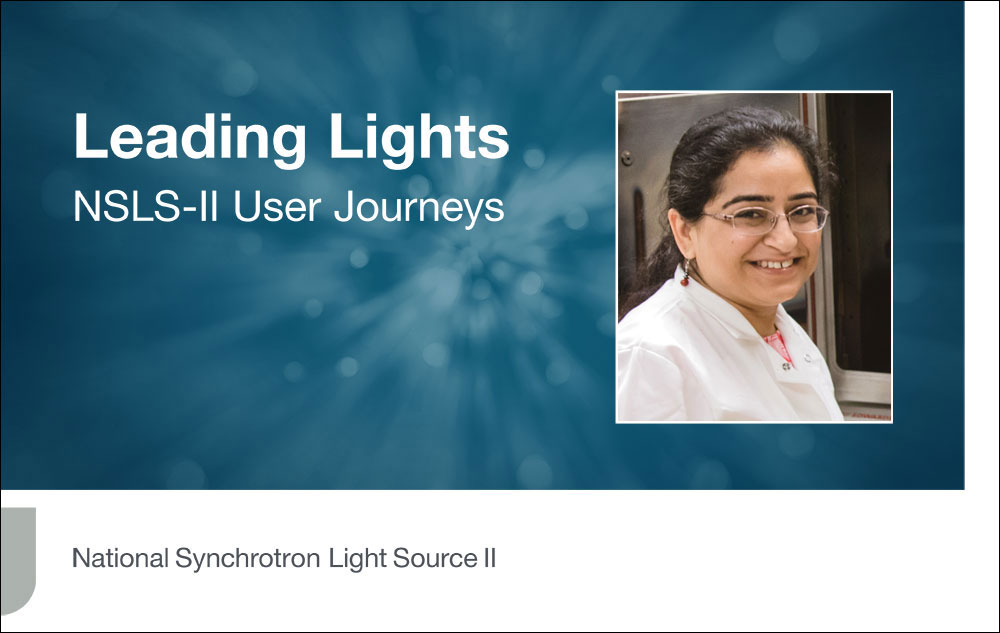
Roopali Kukreja is a professor in the Materials Science and Engineering Department at the University of California, Davis, a public research university. Her research is focused on electronic and magnetic materials with widespread applications, including in quantum computing. To investigate the functional properties of these materials, Kukreja leverages the coherent and highly stable x-ray beams available at the National Synchrotron Light Source II (NSLS-II)—a U.S. Department of Energy (DOE) Office of Science User Facility at DOE’s Brookhaven National Laboratory. Kukreja’s research at NSLS-II is supported in part by a DOE Early Career Award.
What materials do you study and why do they interest you?
My research predominantly focuses on quantum materials. These materials have very interesting magnetic, electronic, and optical properties with exciting applications in quantum computing, energy harvesting, solid state devices, and nonvolatile memory. For most of these applications, you need to control how the material goes from one state to the other. So, my research aims to identify the fundamental changes that occur in these materials as they move between different phases. For example, a material might be electronically conducting at room temperature and insulating at a lower temperature. The polarization of ferroelectric materials can also be controlled by temperature. So, as my research group alters the temperature of these materials, we try to understand how their behavior changes, both at nanoscale length scales and their fundamental time scales.
Why do you choose to come to NSLS-II to study the behavior of complex oxides?
At NSLS-II, I am measuring thermal and quantum fluctuations in materials. You might imagine a solid or a thin film that’s sitting at room temperature as a stable material, but in fact, it has nanoscale domain fluctuations. There can be different things that might be moving around in that sample, for example Brownian motion in liquid, that can define its properties. It’s a very challenging measurement because you need access to nanoscale information, in addition to being sensitive to whatever that domain looks like.
This type of measurement is not something you can do everywhere. There are only four beamlines in the U.S. that are capable of doing this research and two are at NSLS-II. In addition, NSLS-II offers both soft and hard x-rays capable of doing those experiments, so you can measure different aspects or ordering of the same material.
What beamlines at NSLS-II do you most often work with?
The Coherent Hard X-ray Scattering (CHX) beamline and the Coherent Soft X-ray Scattering (CSX) beamline. First, these beamlines at NSLS-II give us a higher intensity of coherent flux. Second, they provide high stability. If you’re measuring fluctuations at room temperature, you need to make sure you’re not picking up fluctuations in your beamline. You need very stable and very precise measurements.
For some routine experiments, we can actually run them ourselves at these beamlines. For more complex experiments, the beamline staff are there to guide us. I cannot thank the beamline staff enough. They work very hard to make some of our most challenging experiments possible. They’ve done measurements late at night for us; they’ve been there 24 hours supporting us. They provide very good feedback on our experimental results and analysis.
Can you give an example of how you’ve used these capabilities in your research?
In one study, we used x-ray photon correlation spectroscopy (XPCS) at CHX to study a model ferroelectric material that our collaborators grew in a new way. They used a different substrate that gave the material different domain structures. The material starts with one ferroelectric domain structure, and then at 45 degrees Celsius, it shows a second domain structure. Then, at 130 degrees Celsius, the material goes through the phase transition completely and changes from ferroelectric to dielectric. So, we wanted to know how the domain structure evolves at room temperature. Is it stable or is it fluctuating like Brownian motion? Are the two transitions occurring in this material compatible? So, we turned to XPCS at NSLS-II.
To understand XPCS, imagine a laser light. If you point the laser onto a rough wall, it will give you an interference pattern, because the light would scatter from the rough surface and interfere with itself. That pattern encodes information about the roughness of the wall. But instead of laser light, we used coherent x-rays through our sample, which gets encoded on a detector. Using this technique at CHX, we found the domains in our material were fluctuating at room temperature. There was an entire 55-degree window near the room temperature where we observed domain fluctuation. When you have fluctuations like this, you can use them as an antenna or use them as a microwave source. More interestingly, you can design it in different configurations so that you can tune the fluctuations to your needs.
What excites you most about this field of research?
The complexity of quantum materials. There is so much unknown. There are so many things tied together and related to each other from nanoscale behavior to macroscopic properties. It excites me how complex these seemingly simple questions can be, such as, “what is the origin of ferroelectric domain fluctuations?” And we still don’t have a convincing answer.
Brookhaven National Laboratory is supported by the Office of Science of the U.S. Department of Energy. The Office of Science is the single largest supporter of basic research in the physical sciences in the United States and is working to address some of the most pressing challenges of our time. For more information, please visit science.energy.gov.
Follow @BrookhavenLab on Twitter or find us on Facebook.
2022-19051 | INT/EXT | Newsroom