Understanding Superconductivity via Atomic Lattice Fluctuations
February 12, 2025
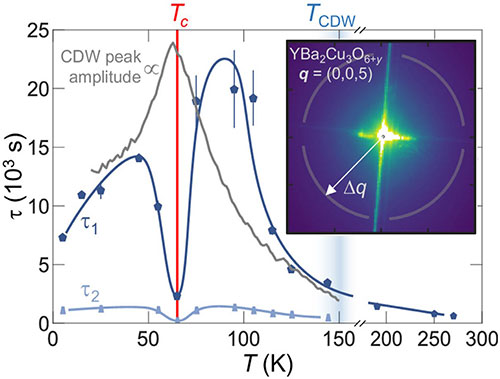
XPCS data show temperature-dependent fluctuation measurements. The CDW peak (gray) is also shown. Inset: diffraction pattern for time-domain measurements collected at 60 K for 5,400 s at a frame rate of 1 Hz.
The Science
Researchers use X-rays to measure slow, subtle fluctuations of the lattice of a high-temperature (Tc) superconductor.
The Impact
Results help elucidate how and why coupling between electrons and the crystal lattice can yield exotic states, such as superconductivity and charge density waves (CDWs).
Summary
The high-temperature superconductors are being extensively studied by research groups across the globe due to their ability to superconduct at temperatures that are much "warmer" — and therefore much more practical — than their standard counterparts. One of the main groups of these materials is the cuprates, which are crystalline compounds based on copper. But scientists still have so much to learn about the intertwined, complex electronic behaviors that occur in these materials, including superconductivity and a state that has been linked to superconductivity, an ordered arrangement of electrons known as a charge density wave (CDW).
These electronic states are not only linked to each other but are also coupled to the material's atomic lattice. But scientists are still working to understand how and why this relationship arises. In a study performed in part at the National Synchrotron Light Source II (NSLS-II), a U.S. Department of Energy (DOE) Office of Science user facility located at DOE's Brookhaven National Laboratory, a group of researchers have uncovered some insight into how the lattice is linked to these special electronic states.
The group used an X-ray technique called X-ray photon correlation spectroscopy (XPCS) to study the slow, subtle fluctuations of the crystal lattice of a common cuprate, YBa2Cu3O6+y (YBCO), which was performed at the NSLS-II Coherent Hard X-ray Scattering beamline. Due to the process of doping — a practice of introducing a small amount of an impurity into a material to produce or enhance certain behavior — the atomic lattice of YBCO does not sit in its perfect atomic positions and is subject to slow motions. The researchers wanted to learn more about how the lattice degrees of freedom and charge are connected, and how those short-range connections could lead to long-range order, such as the CDW.
As the results of the XPCS data were collected from their YBCO sample over thousands of seconds, the group saw that there is a correlation between the speed of the lattice fluctuations and the presence of either a CDW or the superconducting state. In the CDW state, the fluctuations were slower, while they speed up when the material reached its critical superconducting temperature, Tc. This work reveals that measuring lattice fluctuations with bright, coherent X-ray beams can provide spatially resolved details into atomic, as well as charge structure. The results add to the fundamental understanding of how superconductivity, CDWs, and the lattice are coupled.
Download the research summary slide (PDF)
Related Links
Contact
Lingjia Shen
Stanford University,
lingjias@slac.stanford.edu
Stanford University
Joshua J. Turner
joshuat@stanford.edu
Publications
Zach Porter, Lingjia Shen, Rajan Plumley, Nicolas G. Burdet, Alexander N. Petsch, Jiajia Wen, Nathan C. Drucker, Cheng Peng, Xiaoqian M. Chen, Andrei Fluerasu, Elizabeth Blackburn, Giacomo Coslovich, David G. Hawthorn, and Joshua J. Turner. "Understanding the superconductivity and charge density wave interaction through quasi-static lattice fluctuations." Proceedings of the National Academy of Sciences December 4, 2024 121 (50) e2412182121. DOI: https://doi.org/10.1073/pnas.2412182121
Funding
This work is primarily supported by the U.S. Department of Energy, Office of Science, Basic Energy Sciences, Materials Sciences and Engineering Division under Contract No. DE-AC02-76SF00515 through the Early Career Research Program of J.J.T., as well as by the Linac Coherent Light Source, SLAC National Accelerator Laboratory, by the U.S. Department of Energy, Office of Science, Office of Basic Energy Sciences under Contract No. DE-AC02-76SF00515. This research used resources at the CHX beamline of the National Synchrotron Light Source II, a U.S. Department of Energy (DOE) Office of Science User Facility operated for the DOE Office of Science by Brookhaven National Laboratory under Contract No. DE-SC0012704.
2025-22358 | INT/EXT | Newsroom