Q&A with CFN Scientist Anibal Boscoboinik
Using surface-science tools and a 2D model system, Boscoboinik studies zeolite catalysts, which are used in oil refining, plastics production, and nitrogen oxide reduction
December 29, 2016
Materials scientist Anibal Boscoboinik of the Interface Science and Catalysis Group at the Center for Functional Nanomaterials studies the surface chemistry of catalysts at the atomic level. He uses a variety of surface-science tools and techniques—scanning probe microscopy, x-ray photoelectron spectroscopy, and infrared reflection absorption spectroscopy—to characterize the surfaces and interfaces of materials involved in catalysis, in real time and under reaction conditions. His research focuses on heterogeneous catalysis, with a particular interest in nanoporous catalysts such as zeolites, whose surfaces are notoriously difficult to probe.
What is the function of a catalyst, and why is heterogeneous catalysis, as opposed to homogeneous catalysis, the focus of your research?
A catalyst is an agent that changes the mechanism of a chemical reaction such that the conversion of reactants to products typically becomes “easier.” The catalyst does not affect what the reactants and products are; it only changes the rate at which the reaction happens by providing a different pathway for the reaction to occur. In industry, catalysts are used to speed up reactions.
There are two kinds of catalysis: heterogeneous and homogeneous. In heterogeneous catalysis, the catalyst is in a different phase than the reactants and products. Typically, the catalyst is a solid and the reactants and products are gases or liquids. Because of these different phases, it is very easy to separate the products from the catalyst after the reaction—an advantage in industrial processes. In contrast, homogeneous catalysis makes separation very difficult because the catalyst, reactants, and products are all in the same phase.
Why are nanoporous zeolites of particular interest, and what makes probing their surface science so difficult?
Zeolites are crystalline solids made of aluminum, oxygen, and silicon, and they have a cage-like three-dimensional (3D) structure with open pores and channels that are the size of small molecules. There are more than 230 naturally occurring and synthetic zeolites. Many important reactions are catalyzed by these compounds. In fact, they are the most widely used catalysts by volume within industry. In oil refining, zeolites crack the large hydrocarbon molecules of crude oil into smaller molecules that are easier to use as fuels. Cracking catalyzed by zeolites also provides starting materials for a multitude of other chemical processes, including the production of plastics. Toxic nitrogen-oxide emissions from diesel engines are converted into nitrogen through zeolite catalysts. Zeolites also catalyze methanol—a renewable resource—into gasoline. These are just some of the many reactions catalyzed by zeolites.
The nanoporous structure that makes zeolites unique also makes them difficult to study with traditional surface-science tools, which typically require an exposed surface, because the catalytically active surface is enclosed within the pores.
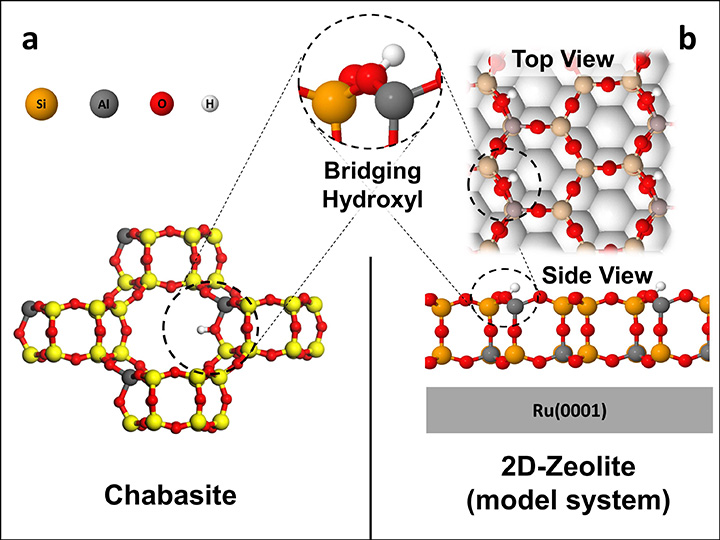
Chabasite (left) is one of the many zeolite frameworks that are used as industrial catalysts. Its catalytically active site is a bridging hydroxyl group. A synthetic 2D zeolite model (right), prepared at CFN, has the same bridging hydroxyl active site, allowing Boscoboinik and his colleagues to study zeolite chemistry on a 2D material.
How do you overcome the challenge of studying zeolites?
My colleagues and I created a two-dimensional (2D) analog material that has the same chemical composition and active site as the 3D porous zeolite. The only difference is that the 2D material’s active site is exposed on a flat surface. With this approach, we can use surface-science tools to probe, down to the atomic scale, the same processes that are happening inside the pores of industrially used zeolites.
Scientists had been trying to make such a 2D model for decades. It was only four years ago, while I was working at the Fritz Haber Institute of the Max Plank Society, that we were able to prepare this “dummy” zeolite material. We showed that this structure has the same chemical behavior as a real zeolite. A few research groups around the world are now using this model.
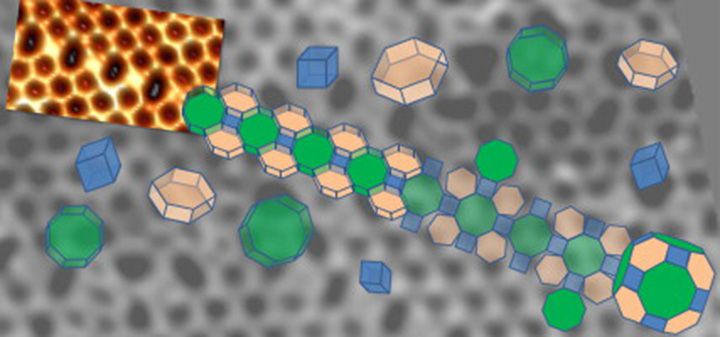
A scanning tunneling microscopy image of the 2D aluminosilicate zeolite model shows polygonal prism units. Aluminum or silicon atoms are located at the vertices of the polygons; oxygen atoms are at the edges. A section of the surface with alternating four- and eight-member rings (polygons) is emphasized and shown in cartoon version. This section can be unfolded and refolded into alpha cages, which are commonly found in zeolites
Can you describe some of the surface-science tools and techniques you employ?
In this research, we most commonly use three techniques: scanning probe microscopy (in particular, scanning tunneling microscopy), x-ray photoelectron spectroscopy, and infrared reflection absorption spectroscopy. The techniques are very complementary, as each one allows us to get a different view of the same surface and potentially a reaction on that surface as it is happening. By probing the catalytically active surface with the scanning tunneling microscope, we get atomic-resolution images that reveal the individual atoms of the catalyst and the molecules that react on it. With x-ray photoelectron spectroscopy, we follow the chemical (oxidation) state of individual atoms on the catalyst, reactants, products, and intermediate species. To study how the atoms are connected, we employ infrared reflection absorption spectroscopy, which allows us to look at the vibrations between atoms. These vibrations tell us what bonds are breaking and forming.
We carry out these techniques in ultrahigh vacuum, and we have been developing instruments to do the same kind of real-time measurements under operating conditions—called operando. Using these instruments, we can study how catalysts work at different pressures and temperatures.
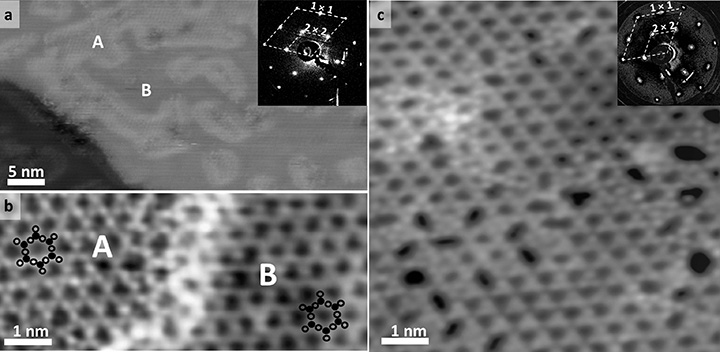
Scanning tunneling microscopy images of 2D zeolite aluminosilicate films (a) at long range, (b) close up, with the location of silicon or aluminum atoms (black circles) and oxygen (open circles) indicated for two of the rings, and (c) containing a higher concentration of aluminum, with crystalline and amorphous regions present on the surface. The insets in (a) and (c) show the low-energy electron diffraction patterns, which reveal the long-range (2 x 2) crystalline ordering of the zeolite model system. Surface domains of different elemental composition are indicated by the capital letters A (silicon, oxygen, and aluminum atoms) and B (only silicon and oxygen atoms).
Do you conduct your research at any other Brookhaven Lab facilities or collaborate with other departments at Brookhaven?
For x-ray photoelectron spectroscopy (XPS), we have an end station at the Soft X-ray Spectroscopy and Polarization (CSX-2) beamline at the National Synchrotron Light Source II (NSLS-II). Here, we conduct ambient-pressure XPS experiments, at relevant pressure and temperature conditions. We are now working jointly with NSLS-II in developing an instrument that simultaneously does ambient-pressure XPS and infrared reflection absorption spectroscopy (IRRAS). There is great synergy between the CFN and NSLS-II user facilities, and we are making great progress by working together. We also collaborate with the Chemistry Department, which has additional ambient-pressure instrumentation and a lot of experience in model catalysis. For the model 2D zeolites, we are also using a reactor scanning tunneling microscope and an IRRAS chamber at the CFN to conduct the high-pressure studies.
What excites you most about your work?
I like being able to play around and explore new ideas within the context of the CFN and larger DOE mission to address the nation’s energy challenges. For example, in our initial work, my colleagues and I explored how catalysts and molecules interact at very low pressures in ultrahigh vacuum. Then we started moving into high-pressure studies. One of our first studies was to see how a 2D zeolite catalyst behaves in the presence of oxygen and hydrogen. We followed the reaction at high pressures and varying temperatures. We found that the catalyst itself does not seem to be affected by either of these gases, but it does affect the chemistry in the confined nanospace between the model catalyst and a metal surface used as support. We wanted to make sure that our 2D zeolite model was structurally and chemically stable so that we can run the reactions without the decomposition of the model catalyst, and while doing that we found new exciting chemistry happening on confined spaces.
Aside from our work on the 2D zeolite model, we are collaborating with a group at the University of Minnesota that has successfully prepared nanosheets of real zeolites. Using their zeolite nanosheet samples, we are exploring how these 2D zeolites interact with methanol, which is very important in the context of methanol-to-gasoline conversion. So far, we discovered that methanol gets trapped inside of the zeolite pores, affecting the vibrations within the zeolite structure.
Our goal with all of these studies is to learn more about how catalysts operate. Most if not all of the industrial catalysts are made to a great extent by trial and error—in a way, by mixing materials and testing if they work. Industry has now reached the point where a more rational way of preparing catalysts is needed. The new philosophy is catalyst by design. For this philosophy, we need design guidelines. But first we need to know how catalysts work. We still do not know a great deal about the mechanistic aspects of catalysis in many cases. The very sensitive surface-science tools we are using will help uncover these mechanisms.
Have you encountered any surprise discoveries along the way?
Probably half of the results I have published came from unexpected findings along the way. Actually, the study I just described in which we tested how a catalyst responds to the presence of hydrogen or oxygen resulted in a surprise discovery. In this study, we had the catalyst sitting on top of ruthenium, a transition metal. We noticed that ruthenium, which normally oxidizes very easily in the presence of oxygen, had very different behavior when the 2D zeolite was on top of it. The ruthenium surface remained unchanged in the presence of oxygen at elevated temperatures. So, in this case, the zeolite was not only a catalyst but also an anti-corrosion coating!
In another study, we were looking at a 2D zeolite structure during instrument testing. We introduced an inert gas (argon) and thought nothing would happen in the high-pressure chamber. But to our surprise, argon got inside of the structure’s “cages” and stayed trapped in the pores. Usually, argon condenses at a very low temperature (40 Kelvin), yet this happened at room temperature (300 Kelvin). When we heated the structure, the argon was released. We then tried krypton and xenon—two nuclear waste products generated by power plants—which also got trapped inside the cages. It took a very high temperature to release these gases. So, we were conducting catalysis research and ended up with a finding that may have an impact in nuclear waste remediation—a potential way to trap these volatile radioactive gases.
Anibal Boscoboinik discusses his research on zeolites.
What brought you to the Center for Functional Nanomaterials?
When I applied for the position, I was in Germany, doing postdoctoral work at the Fritz Haber Institute of the Max Planck Society. I really wanted to come back to the United States, where I did my PhD in chemistry, because of the diversity and open-mindedness I encountered within the academic and research environment. I knew that CFN was one of the leading centers in the world for nanotechnology research. Things worked out well!
The Center for Functional Nanomaterials hosts users from industry, universities, and government laboratories around the world. How has your immersion in this environment impacted you, personally and professionally?
Working at the CFN has been great from both a cultural and research point of view. I am originally from Argentina, and I have previously worked with scientists from all different countries, so continuing those interactions at CFN has been enjoyable. The CFN community is full of different research backgrounds and ways of thinking. Working at a user facility helps you open your mind to what other scientists are researching—something that we do not always do as scientists because we are so focused on our own specific research problems. When you have the tree right in front of you, it is very easy to lose track of the forest. At the CFN, we get exposure to research projects other than our own and benefit from cross-disciplinary collaborations. For example, we work with scientists in the CFN’s Theory and Computation Group who use theoretical modeling to make sure our experimental structures are energetically feasible.
Mostly, we collaborate with universities—I am currently co-mentoring two doctoral students from Stony Brook University—and other national lab–like institutions in other countries. Industry collaborations are a work in progress, as we are only at the very basic research stage.
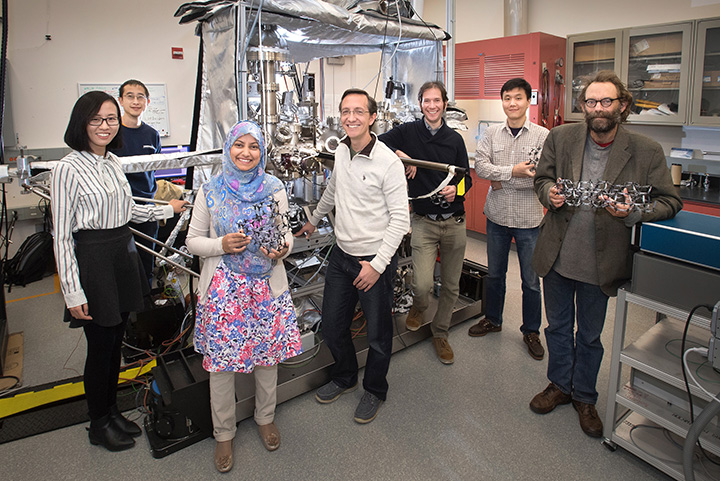
(Left to right) Mengen Wang, Deyu Lu, Nusnin Akter, Dario Stacchiola, Anibal Boscoboinik, JianQiang Zhong, and John Kestell in one of CFN's characterization labs, where they use a scanning tunneling microscope to follow the catalytic processes happening in their 2D zeolite model, with atomic resolution.
When and how did you become interested in chemistry and specifically catalysis?
When I went to college, I enjoyed science and engineering in general. Chemistry seemed like a good compromise because I could apply many different aspects of science that I like, particularly physics (physical chemistry is what I am doing now) and mathematics. After graduating from college, I went to work in industry and did not like it. I moved to the United States to pursue my PhD in chemistry. The research group I landed in was doing catalysis research at the time, and I found their work in heterogeneous catalysis very interesting. I joined them, and I have been studying catalysis ever since. The fact that we can understand in so much detail how each individual atom interacts keeps me wanting to learn more.
2016-6754 | INT/EXT | Newsroom