Synthesized Hierarchical Structures in Solid-state Chemistry
March 20, 2014
Traditional solid-state compounds are made up of individual atoms arranged in crystalline arrays in three dimensions. But technological progress and creativity led researchers to try a new way of making solid-state materials.
Their new materials mimic atomic structures, but instead of making solids from atoms, they made them with molecules that stand in for the atoms. These molecular clusters are larger than atoms and they produce collective properties, such as electrically conducting networks and magnetic ordering.
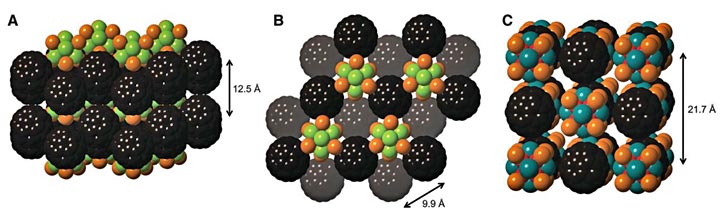
Space-filling structure of solid-state compound 1•2C60, showing the crystal packing looking along (A) the ab plane and (B) down the c axis. (C) Space-filling structure of 3•C60. Carbon, black; nickel, red; cobalt, blue; phosphorus, orange; tellurium, teal; selenium, green. The ethyl groups on the phosphines were removed to clarify the view.
“In the solid-chemistry business, you’re limited to the naturally occurring atom if you want to make solids,” said research scientist Michael Steigerwald of Columbia University. “And you can’t make new atoms. But if you think about it this way, we can make new atoms by making new clusters. And the hope or expectation is that we’ll be able to make new types of materials with new and useful properties if we build them up with molecules that we can make and assemble.”
Of the three solid-state materials they created, two are similar in lattice structure to cadmium iodide, and one is a simple rock-salt crystal. And they are all electrically conductive. Roy says he and his colleagues have been working with one of the building blocks of these materials – fullerene, or C60, a carbon molecule shaped like a soccer ball – for decades, and only recently decided to combine it with other molecular clusters.
“We knew that C60 likes to accept electrons, and that metal calcitrinide clusters give up electrons. We thought, ‘Gee what happens if we put this electron-rich thing together with this electron-accepting thing?’” he said.
The result was new solid-state metals. “Does the world need a new metal? Maybe not, but a new metal that has a lot of other angles to it, that could be useful. On top of being solid-state, they could potentially absorb light differently.”
Now, said Steigerwald, they will study the ways these materials can be manipulated to produce different properties. Compressing the solids and creating a denser molecular lattice structure or swapping in different elements – say, switching cobalt and nickel for other metals – could lead to new materials.
“There’s a wide range of things to change here, and we’ve just started to get into that,” he said. “Our imagination is the only limit to the different things we can try.”
Researchers from Columbia University, Florida State University, and Stony Brook University contributed to this work.
This work was supported primarily through the Center for Re-Defining Photovoltaic Efficiency Through Molecular-Scale Control, an Energy Frontier Research Center funded by the U.S. Department of Energy.
2014-4726 | INT/EXT | Newsroom