Closing in on the Border Between Primordial Plasma and Ordinary Matter
Energy scan at the Relativistic Heavy Ion Collider (RHIC) reveals first hints of phase boundary
August 13, 2012
UPTON, NY — Scientists taking advantage of the versatility and new capabilities of the Relativistic Heavy Ion Collider (RHIC), an atom smasher at the U.S. Department of Energy’s Brookhaven National Laboratory, have observed first glimpses of a possible boundary separating ordinary nuclear matter, composed of protons and neutrons, from the seething soup of their constituent quarks and gluons that permeated the early universe some 14 billion years ago. Though RHIC physicists have been creating and studying this primordial quark-gluon plasma (QGP) for some time, the latest preliminary data—presented at the Quark Matter 2012 international conference—come from systematic studies varying the energy and types of colliding ions to create this new form of matter under a broad range of initial conditions, allowing the experimenters to unravel its intriguing properties.
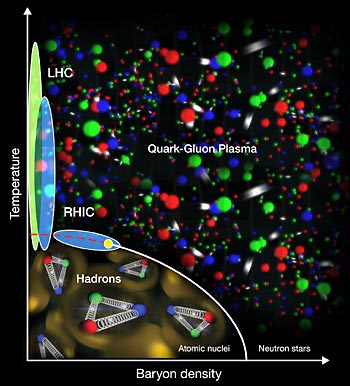
The nuclear phase diagram: RHIC sits in the energy "sweet spot" for exploring the transition between ordinary matter made of hadrons and the early universe matter known as quark-gluon plasma.
“2012 has been a banner year for RHIC, with record-breaking collision rates, first collisions of uranium ions, and first asymmetric collisions of gold ions with copper ions,” said Samuel Aronson, Director of Brookhaven National Laboratory. “These unique capabilities demonstrate the flexibility and outstanding performance of this machine as we seek to explore the subtle interplay of particles and forces that transformed the QGP of the early universe into the matter that makes up our world today.”
The nuclei of today’s ordinary atoms and QGP represent two different phases of matter whose constituents interact through the strongest of Nature’s forces. These interactions are described by a theory known as quantum chromodynamics, or QCD, so scientists sometimes refer to the exploration of QGP and this transition as the study of QCD matter.
As in other forms of matter, the different phases exist under different conditions of temperature and density, which can be mapped out on a “phase diagram,” where the regions are separated by a phase boundary akin to those that separate liquid water from ice and from steam. But in the case of nuclear matter, scientists still are not sure where to draw those boundary lines. RHIC is providing the first clues.
“RHIC is well positioned to explore QCD phase structure because we can vary the collision energy over a wide range, and in so doing, change the temperature and net quark density with which QCD matter is formed,” said Steven Vigdor, Brookhaven’s Associate Laboratory Director for Nuclear and Particle Physics, who leads the RHIC research program.
For example, physicists from RHIC’s STAR and PHENIX collaborations have analyzed results from gold ion collisions taking place at energies of 200 billion electron volts (GeV) per pair of colliding particles, all the way down to 7.7 GeV.
While at the highest energies evidence for QGP formation is widely accepted, “many of the signatures of the QGP developed at 200 GeV disappear as the energy decreases,” said STAR spokesperson Nu Xu, a physicist at Lawrence Berkeley National Laboratory.
In particular, the STAR findings analyzed so far indicate that interactions among “free” quarks and gluons—those characteristic of the “perfect” liquid QGP discovered at RHIC—appear to dominate at energies above 39 GeV, while at energies below 11.5 GeV, the interactions of bound states of quarks and gluons known as hadrons (such as the protons and neutrons of ordinary matter) appear to be the dominant feature observed.
“As you get below 39 GeV, several key observables begin to change,” Xu said.
The PHENIX experiment has observed similar behavior. They have found that quarks passing through the matter produced at collision energies from 39 GeV upward lose energy rapidly, as anticipated for interactions within QGP. Previous PHENIX results from copper-copper collisions at 22 GeV, in contrast, are consistent with no significant energy loss.
These measurements are helping scientists plot definitive points, or signposts, which tell them they may be approaching the boundary between ordinary nuclear matter and the QGP that dominated the early universe. But they haven’t yet proven that a sharp boundary line exists, or found the “critical endpoint” at the termination of that line.
“The critical endpoint, if it exists, occurs at a unique value of temperature and density beyond which QGP and ordinary matter can co-exist,” said Vigdor. It is analogous to a critical point beyond which liquid water and water vapor can co-exist in thermal equilibrium, he said.
RHIC Explores Matter at the Dawn of Time
Because of the complexity of QCD calculations, there is as yet no consensus among theorists where the QCD critical point should lie or even if it exists. But RHIC experimentalists say they see hints in the data around 20 GeV that resemble signatures predicted to be observed near such a QCD critical point. However, much more data from future experiment runs at RHIC is required to turn these hints into conclusive evidence.
Apparent symmetry violations disappear at low energy
One signal that disappears in gold-gold collisions at RHIC energies below 11.5 GeV is the indication of a small separation of positive from negative electric charge within the matter produced in each individual collision. Ordinarily, such a charge separation would be forbidden by the “mirror symmetry” that is a fundamental feature of QCD. But at the ultra-high temperatures of QGP, the theory allows such symmetry violations to occur in localized “bubbles,” as long as they average out to zero when bubbles from all collision events are looked at together.
“Such symmetry-violating bubbles are of crucial interest in the early, high-temperature history of the universe, where analogous bubbles are speculated to have played a central role in producing the preponderance of matter over antimatter in today’s universe, enabling our existence,” Vigdor said.
The disappearing hints of charge separation may be another signal that the lower-energy RHIC collisions are no longer producing QGP. But it’s also conceivable that the hints arise instead from a “background” phenomenon that is related to the almond-like shape of the overlap region formed when two spherical gold ions collide in not quite head-on fashion.
Head-on collisions of football-shaped uranium ions aligned in upright positions like footballs set for kick-off—conducted for the first time during the 2012 RHIC run, and made possible by a new ion source at RHIC—are allowing scientists to study the effects of this almond-like interaction region without the strong surrounding magnetic field also produced in the off-center gold-gold collisions (which is necessary for the interesting charge-separation signal).
Results so far, reported by STAR physicists at Quark Matter 2012, seem to rule out the role of the background effect. If subsequent analysis confirms this early finding, the uranium-uranium collisions will provide further evidence for the symmetry-violating bubble interpretation of the gold-gold data, and for the disappearance of QGP at the lower RHIC energies.
From ordinary matter to plasma
The way quarks and gluons are arranged in ordinary matter affects how the plasma forms, and also modifies production of experimental probes of the plasma’s properties. Teasing out effects of the plasma on these probes requires good knowledge of the probes before they encounter QGP.
To get that important information, the RHIC experiments have collected a large data set from collisions of gold ions with deuterons (the nuclei of heavy hydrogen).
At Quark Matter 2012, PHENIX physicists report that there are fewer high-momentum single hadrons and collections of hadrons called “jets” produced in dead-on central deuteron-gold collisions than more glancing deuteron-gold collisions.
“We expect jet suppression in quark-gluon plasma, because jets lose energy in dense matter such as the plasma,” said PHENIX spokesperson Barbara Jacak, a physicist at Stony Brook University. “But this result shows that we have to correct for this initial state effect when figuring out how much the plasma suppresses the production of jets.”
The initial state is related to the arrangement of quarks and gluons deep inside the gold nucleus, which some theories predict could be a condensed form of gluons called color-glass condensate, as hinted at in earlier results published by PHENIX.
The force between quarks and antiquarks
Other new RHIC measurements reported at Quark Matter concern the probability of heavy quarks (bottom and charm) and their anti-matter counterparts pairing up to form bound states called “quarkonia” within the QGP and in the “cold” nuclear matter probed in the deuteron-gold collisions.
QCD tells us that the force between a quark and an antiquark increases in strength as they are pulled apart, as though they were connected by an invisible rubber band. But the strength of this force should be reduced in QGP. So physicists expect the formation of quarkonia to also be reduced in QGP, with the probability of finding such species decreasing with larger-size bound states.
The STAR experiment reported new results consistent with this expectation by studying different size bound states of bottom quarks and antiquarks. PHENIX has studied suppression of bound states of charm and anti-charm quarks in various beam combinations, both with and without plasma formation. New results indicate that their formation is already suppressed in collisions of deuterons with gold nuclei, when no QGP is formed.
“This reflects both the reduced production rates for heavy quarks and the fact that the bound state sometimes breaks up as it passes through normal (cold) nuclear matter,” said Jacak. “It is crucial to quantify this if we are to understand QGP effects on the binding,” she said.
“These new results on the phase boundary, symmetry-violating bubbles, initial state effects, and the production of quark-antiquark bound states illustrate how scientists are exploiting RHIC’s unique versatility for precision determinations of the properties of quark-gluon plasma,” Vigdor said. “It is this versatility, in combination with dramatic advances we’ve made in the rate of collisions provided at RHIC, that will allow our scientists in the coming decade to answer the pointed questions raised by RHIC’s exciting discoveries about this early universe matter.”
Research at RHIC is funded primarily by the DOE Office of Science, and also by these agencies and organizations.
DOE's Office of Science is the single largest supporter of basic research in the physical sciences in the United States, and is working to address some of the most pressing challenges of our time. For more information, please visit science.energy.gov.
2012-11446 | INT/EXT | Newsroom