Perfecting the View on a Crystal's Imperfection
New research shines light on the properties and promise of hexagonal boron nitride, a material used in electronic and photonics technologies
April 23, 2024
By Shawn Rhea
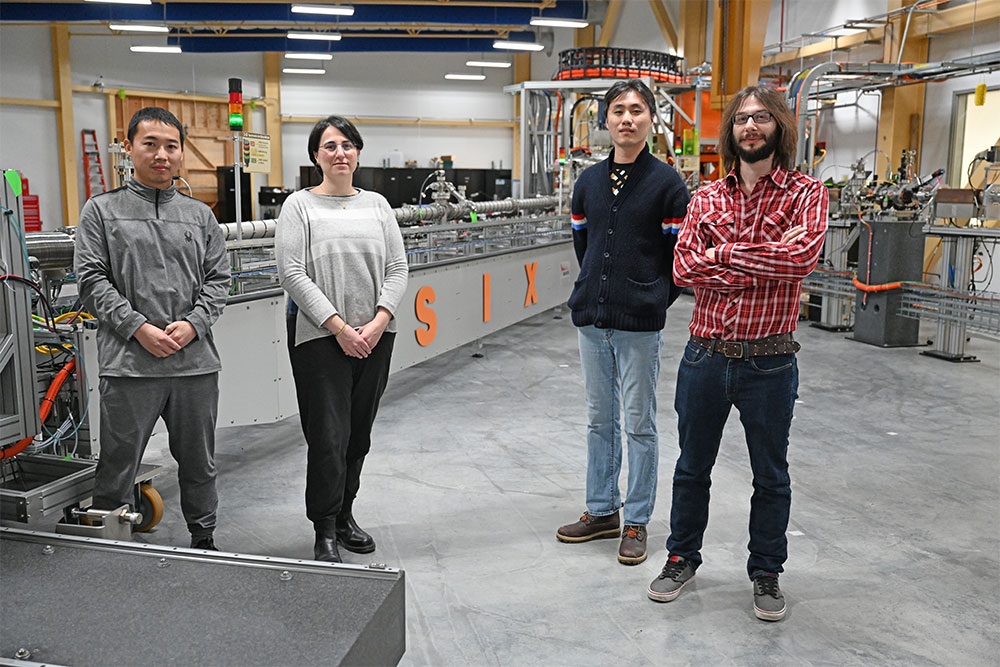
From left to right: NSLS-II scientists Jiemin Li, Valentina Bisogni, Shiyu Fan, and Jonathan Pelliciari at the SIX beamline. (Kevin Coughlin/Brookhaven National Laboratory)
Editor's note: The following press release was originally issued by the City University of New York (CUNY). This research was co-led by Gabriele Grosso, an assistant professor of physics at CUNY, and Jonathan Pelliciari, a beamline scientist at the at the National Synchrotron Light Source II (NSLS-II), a U.S. Department of Energy (DOE) Office of Science User Facility at DOE’s Brookhaven National Laboratory. The team used the Soft Inelastic X-ray Scattering (SIX) beamline at NSLS-II to uncover distinctive quantum emissions caused by defects in the structure of hexagonal boron nitride. For more information on Brookhaven’s role in this research, contact Denise Yazak (dyazak@bnl.gov, 631-344-6371).
NEW YORK — Single-photon emitters (SPEs) are akin to microscopic lightbulbs that emit only one photon (a quantum of light) at a time. These tiny structures hold immense importance for the development of quantum technology, particularly in applications such as secure communications and high-resolution imaging. However, many materials that contain SPEs are impractical for use in mass manufacturing due to their high cost and the difficulty of integrating them into complex devices.
In 2015, scientists discovered SPEs within a material called hexagonal boron nitride (hBN). Since then, hBN has gained widespread attention and application across various quantum fields and technologies, including sensors, imaging, cryptography, and computing, thanks to its layered structure and ease of manipulation.
The emergence of SPEs within hBN stems from imperfections in the material’s crystal structure, but the precise mechanisms governing their development and function have remained elusive. Now, a new study published in Nature Materials reveals significant insights into the properties of hBN, offering a solution to discrepancies in previous research on the proposed origins of SPEs within the material. The study involves a collaborative effort spanning three major institutions: the Advanced Science Research Center at the CUNY Graduate Center (CUNY ASRC); the National Synchrotron Light Source II (NSLS-II) user facility at Brookhaven National Laboratory; and the National Institute for Materials Science. Gabriele Grosso, a professor with the CUNY ASRC’s Photonics Initiative and the CUNY Graduate Center’s Physics program, and Jonathan Pelliciari, a beamline scientist at NSLS-II, led the study.
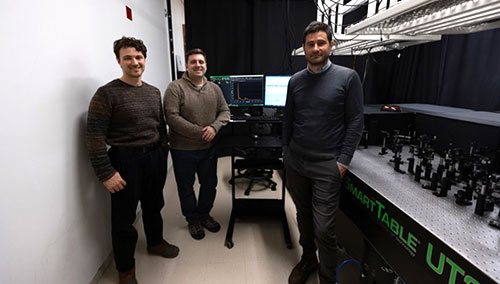
Researchers in the labs of ASRC Prof. Gabriele Grosso (top, right) and NSLS-II at Brookhaven National Laboratory used advanced instrumentation at their respective facilities to uncover how single photon emitters, which have important used in quantum technologies, emerge within hexagonal boron nitride crystals. (Shante Booker/CUNY ASRC)
The collaboration was sparked by a conversation at the annual NSLS-II and Center for Functional Nanomaterials Users’ Meeting when researchers from CUNY ASRC and NSLS-II realized how their unique expertise, skills, and resources could uncover some novel insights, sparking the idea for the hBN experiment. The work brought together physicists with diverse areas of expertise and instrumentation skillsets who rarely collaborate in such a close manner.
Using advanced techniques based on X-ray scattering and optical spectroscopy, the research team uncovered a fundamental energy excitation occurring at 285 millielectron volts. This excitation triggers the generation of harmonic electronic states that give rise to single photons — similar to how musical harmonics produce notes across multiple octaves.
Intriguingly, these harmonics correlate with the energies of SPEs observed across numerous experiments conducted worldwide. The discovery connects previous observations and provides an explanation for the variability observed in earlier findings. Identification of this harmonic energy scale points to a common underlying origin and reconciles the diverse reports on hBN properties over the last decade.
“Everyone was reporting different properties and different energies of the single photons that seemed to contradict each other,” said Grosso. “The beauty of our findings is that with a single energy scale and harmonics, we can organize and connect all of these findings that were thought to be completely disconnected. Using the music analogy, the single photon properties people reported were basically different notes on the same music sheet.”
While the defects in hBN give rise to its distinctive quantum emissions, they also present a significant challenge in research efforts to understand them.
“Defects are one of the most difficult physical phenomena to study, because they are very localized and hard to replicate,” explained Pelliciari. “Think of it this way; if you want to make a perfect circle, you can calculate a way to always replicate it. But if you want to replicate an imperfect circle, that’s much harder.”
The implications of the team’s work extend far beyond hBN. The researchers say the findings are a stepping stone for studying defects in other materials containing SPEs. Understanding quantum emission in hBN holds the potential to drive advancements in quantum information science and technologies, facilitating secure communications and enabling powerful computation that can vastly expand and expedite research efforts.
"These results are exciting because they connect measurements across a wide range of optical excitation energies, from single digits to hundreds of electron volts,” said Enrique Mejia, a Ph.D. student in Grosso lab and lead author of the work conducted at the CUNY ASRC. “We can clearly distinguish between samples with and without SPEs, and we can now explain how the observed harmonics are responsible for a wide range of single photon emitters."
This work was funded by LDRD, FWP DOE on quantum information science, DOE BES, and DOE ECA. The work at CUNY was supported by the National Science Foundation (NSF), the CUNY Graduate Center Physics Program, the CUNY Advanced Science Research Center, and the CUNY Research Foundation.
About the Advanced Science Research Center at the CUNY Graduate Center
The Advanced Science Research Center at the CUNY Graduate Center (CUNY ASRC) is a world-leading center of scientific excellence that elevates STEM inquiry and education at CUNY and beyond. The CUNY ASRC’s research initiatives span five distinctive, but broadly interconnected disciplines: nanoscience, photonics, neuroscience, structural biology, and environmental sciences. The center promotes a collaborative, interdisciplinary research culture where renowned and emerging scientists advance their discoveries using state-of-the-art equipment and cutting-edge core facilities.
About Brookhaven National Laboratory
Brookhaven National Laboratory is supported by the Office of Science of the U.S. Department of Energy. The Office of Science is the single largest supporter of basic research in the physical sciences in the United States and is working to address some of the most pressing challenges of our time. For more information, visit science.energy.gov.
Follow @BrookhavenLab on social media. Find us on Instagram, LinkedIn, X, and Facebook.
2024-21842 | INT/EXT | Newsroom