iCFN User Spotlight—Latha Venkataraman: Advancing the World's Smallest Devices
December 11, 2013
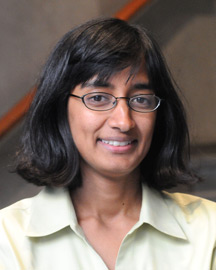
Latha Venkataraman
Latha Venkataraman, physicist and Associate Professor at Columbia University, specializes in sensitive nanoscale systems that could one day form the foundation for technological breakthroughs. Understanding how these devices work, and optimizing their function requires instruments with extraordinary precision and cutting-edge computational expertise—the latter brought Venkataraman to Brookhaven National Laboratory’s Center for Functional Nanomaterials.
What compels you to explore nanoscale electronic and mechanical devices?
The smallest electronic devices imaginable still need a few atoms to operate. By developing circuits with components that consist of a single molecule—a collection of a few atoms—I am working at a size regime that is close to the fundamental limit. There’s something exciting about exploring how things work at such an extreme and largely uncharted scale. If we understand the electronics at the single-molecule level, that knowledge may help optimize larger devices and organic-based electronics and photovoltaics.
How do these molecular devices fit into the pursuit of improved energy efficiency?
A lot of the electricity we use comes from highly inefficient processes that generate heat as waste, from coal-burning power plants to vehicle engines. Improving our ability to convert this wasted heat to electricity could have a huge technological impact. One mechanism for this process is the thermoelectric effect in which a temperature difference between two sides of a device can induce a voltage drop across it. Unfortunately, the efficiency for this heat-to-electricity conversion mechanism has been low. To understand how to increase this efficiency, we study thermoelectric devices that consist of single molecules connected to two metal wires. To put it simply, we want to maximize the current that flows through the single-molecule device in response to an imposed temperature difference. In this recent Nano Letters study, we found that the conversion efficiency was maximized by creating a single-molecule junction using an all-carbon molecule.
Why bring that research to Brookhaven Lab’s Center for Functional Nanomaterials?
The short answer is that Mark Hybertsen, a physicist at CFN, is a fantastic collaborator with the ideal set of skills and expertise to complement my work. Mark specializes in using theory and computation to both guide and interpret materials science. I do most of my experimental work using instruments at Columbia University, but Mark plays an essential role in making sense of our findings. CFN offers a very compelling package for someone working on very complex systems—I have access to a leading expert, custom-designed software, and a powerful computing cluster. Mark and I actually met as office mates at Columbia years before he joined Brookhaven Lab, and we continue to collaborate closely.
How did you work together to improve the performance of your single-molecule thermoelectric device?
Well, down on the molecular scale you can play tricks with quantum mechanics to create that optimal balance. We found in an earlier work that we can maximize the conductance (how easily current flows in response to voltage) of a single-molecule device by using all-carbon molecules that are covalently bonded to the metal electrodes, without using any chemical “alligator clips” that typically end up creating a bottleneck for current flow. We carried out these measurements in a custom scanning tunneling microscope (STM) developed at Columbia. We then suspected that these all-carbon molecules had potential for thermoelectric devices, which was indeed what we found from the experiments. However, the question remained: Why does it work so well?
Mark led full calculations on the device’s performance, which are rather complicated to carry out, with a joint post-doctoral researcher. However, these did not give us an intuitive understanding of our results or how the trends in our measurements could be used to design better devices. Mark felt that we would learn more from a simpler model calculation that my graduate student, who did the measurements, could also carry out. The simple toy model actually predicted the experimental results and allowed us to understand how carbon-gold covalent bonds were the key to obtaining the higher thermoelectric efficiencies. More importantly, it provided a rather simple tool for all my graduate students to use and learn from.
What’s next for the development of single-molecule devices?
One of the goals of this research is to create molecular devices with varied functionalities that are controlled through chemical design. In a recent study motivated by this work, we were able to apply that new theoretical model to a different experiment designed to study rectification (diode behavior) at the single-molecule level. Because of our ability to control the bond between gold and carbon, we showed that we could tune the diode performance through precise chemistry.
Another significant question hangs over working with these nanoscale devices: Is it possible to make a molecular device that survives outside of a tightly controlled experimental system? Our junctions have a lifetime measured in milliseconds, and it will take considerable work to make the technology more durable and versatile.
Are there any other recent experiments where you used CFN facilities?
I recently completed a study on single-molecule van der Waals interactions at metal/organic interfaces—these interactions describe the relationship between chemical compounds and surface textures. For example, van der Waals interactions explain how a gecko can cling upside down to a glass ceiling. Beyond that, they impact energy sciences including catalysis, molecular electronics, and material self-assembly.
Modeling van der Waals interactions from first principles is computationally very challenging, but with Mark we were able to model and explain our measurements showing that van der Waals interactions were essential in the single-molecule devices. The science itself is completely different from the thermoelectric study, but CFN’s combo of software, a high-end computing cluster, and theoretical expertise was still the perfect match.
How would you characterize the CFN research community and culture?
Any experimentalist interested in modeling complex reactions will need a computer cluster and an expert collaborator. What sets Mark Hybertsen and CFN apart is that scientific excellence is combined with a genuine interest in the users’ research goals. My students also work with Mark to develop and run these theoretical models, and he is able to tune his interactions to each student’s level of understanding. Finding such flexible and accommodating scientists is rare enough without even considering the top-tier computational facilities.
Latha Venkataraman is an Associate Professor of Applied Physics and Applied Mathematics at Columbia University, which she first joined as a research scientist in 2003. She received her B.S. in Physics at the Massachusetts Institute of Technology and then completed both her M.S. and Ph.D. at Harvard University.
2013-4511 | INT/EXT | Newsroom