


Black Holes at RHIC?
Further discussion by Physicist Dmitri Kharzeev on why RHIC cannot produce a real gravitational black hole
Black holes are among the most mysterious objects in the universe. The gravitational field of a black hole is so strong that Einstein’s general relativity tells us that nothing, not even light, can escape from the black hole’s interior.
However, in 1974 physicist Stephen Hawking demonstrated that black holes must emit radiation once the quantum effects are included. According to quantum mechanics, the physical vacuum is bubbling with short-lived virtual particle-antiparticle pairs. Creation of a particle-antiparticle pair from the vacuum conflicts with energy conservation, but energy need not be conserved at short times in quantum mechanics, according to Heisenberg’s uncertainty principle. The gravitational field of a black hole can resolve the intrinsic structure of the particle-antiparticle pair, and can therefore “suck in” one of the particles of the pair while the second particle is liberated and escapes.
The escaping particles have a thermal spectrum, reflecting the fact that no information can be extracted from the black hole (this latter assertion, however, is a subject of a lively debate at present — it may be possible that a spectrum does contain a small non-thermal correction, meaning that some information can be carried away from the black hole). The temperature of the thermal spectrum appears equal to the acceleration of gravity at the surface of the black hole divided by 2π. Einstein’s Equivalence Principle states that gravity is equivalent to acceleration in a non-inertial frame — and each of us has tested this principle by stepping into an elevator: Being in an accelerating elevator is equivalent to experiencing a stronger gravitational field.
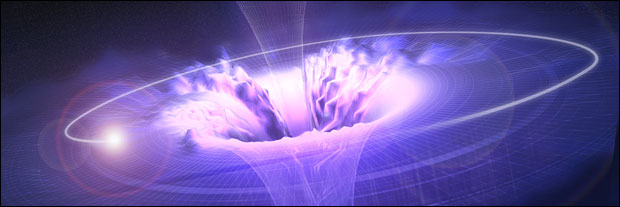
Therefore, the thermal radiation with a temperature equal to the acceleration divided by 2π should be a general feature of all processes accompanied by acceleration (or deceleration). Indeed, this was proved by physicist William Unruh in 1976.
What are the processes that accompany the largest deceleration that can be studied on Earth? The gravitational field of Earth is too weak, and leads to an unobservable thermal temperature of 10-20 degrees Kelvin (K). Electromagnetic fields also appear not strong enough. It appears that, at present, the strongest deceleration achievable in a laboratory is obtained in relativistic heavy ion collisions, where a sizable fraction of quarks and gluons is decelerated from the velocity of light down to zero velocity in a very short distance, about 0.2 femtometers. This deceleration thus must be accompanied by a burst of a hot thermal radiation with a temperature about 200 million electron-volts (MeV), or 1012K (which is far hotter than the Sun, which has a temperature of 107 K).
A rapid deceleration also induces phase transitions in strongly interacting matter, similar to the ones that are believed to occur in the vicinity of a black hole. Indeed, a burst of hot thermal radiation of quarks and gluons has been observed at RHIC, where the abundance of the produced hadrons (hadrons are particles made of quarks and gluons) follow the predictions of thermodynamics, and there is growing evidence of a phase transition to the quark-gluon plasma.
Perhaps the greatest puzzle of RHIC physics is how this thermalization can occur so fast. The “black hole thermalization” scenario naturally explains this fact and provides a set of further predictions that can be tested at RHIC.
Needless to say, the risk of producing a real black hole in RHIC experiments is not higher than during our daily rides in elevators — this danger simply does not exist.