Prototyping the Next Generation
A new, compact accelerator design decreases emittance for an even brighter beam
May 20, 2025
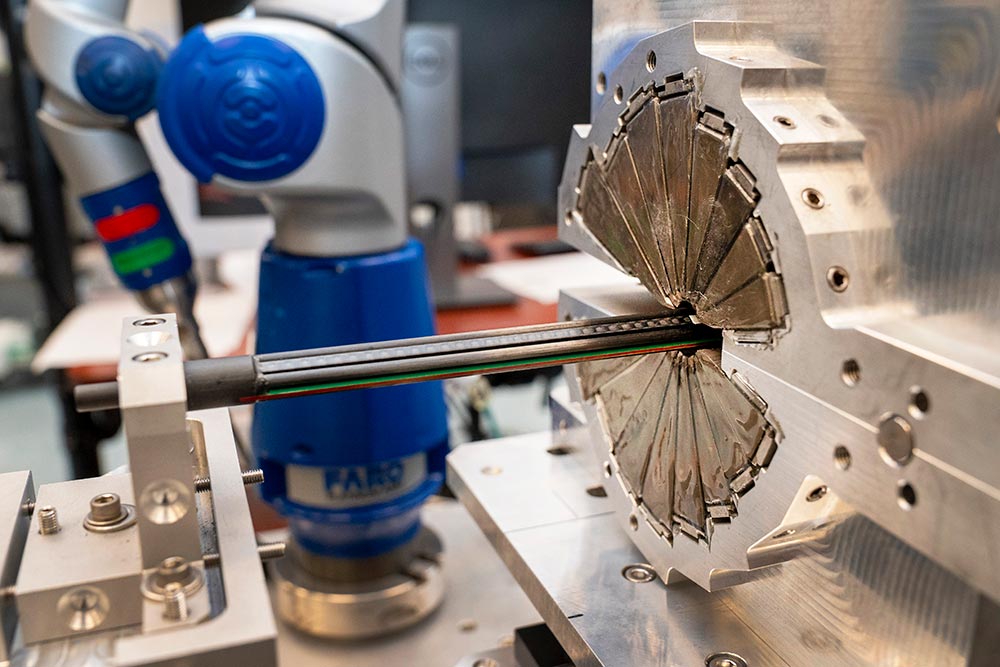
In the National Synchrotron Light Source II Project Research and Development Facility, a prototype permanent magnet quadrupole is being tested for field quality. (David Rahner/Brookhaven National Laboratory)
Researchers at the National Synchrotron Light Source II (NSLS-II), a U.S. Department of Energy (DOE) Office of Science user facility at DOE’s Brookhaven National Laboratory, depend on the facility’s bright, stable electron beam to carry out groundbreaking experiments. Behind the scenes, a dedicated team of physicists, engineers, designers, and technicians in the facility’s accelerator complex are not only maintaining this system for reliable operation but also looking into ways to improve performance and unlock new areas of synchrotron science for the light source’s research community. In an inventive new design that has been years in the making, the team has unveiled a proof-of-principle prototype for a new “complex bend” lattice design. This unique magnet array has sparked discussion about some intriguing possibilities for the future of NSLS-II’s accelerator, and the design is lighting the way for necessary next steps.
Building on a legacy of accelerator innovation
Synchrotrons like NSLS-II use powerful magnets to steer and focus an ultrabright beam of electrons as the beam circulates around a circular track called a storage ring. This beam produces intense X-rays that are directed to experimental stations known as beamlines, where they are used for a wide range of scientific investigations. For decades, light sources around the world have relied on the Chasman-Green lattice, a complex arrangement of magnets also known as the double bend achromat lattice. Developed in the 1970s by Brookhaven Lab scientists Renate Chasman and George Kenneth Green, this design has become a foundational element of modern synchrotron light sources. The NSLS-II storage ring is currently comprised of 30 double-bend achromat cells that support the construction of nearly 60 beamlines. At this time, 29 beamlines are operational and several more are in various stages of development.
In 2018, a group of scientists in NSLS-II’s Accelerator Division, led by Division Director Timur Shaftan, began exploring a new design for an upgraded lattice that showed promise in achieving very strong localized focusing and minimized beam emittance. Emittance is the measurement of how much the electrons in a beam will spread, which is important to light source facilities. The tighter the beam is, the brighter it will be. For this reason, accelerator physicists are on the lookout for ways to minimize emittance as much as they can. This quest helped motivate a novel “complex bend” lattice, which requires closely spaced, high-strength, combined-function magnets. These magnets are placed along a curved trajectory, known as a “Halbach” cylinder arrangement, which requires the use of compact permanent magnet quadrupoles (PMQs). These magnets would serve a combined function, meaning they would operate not only as a quadrupole but also as a dipole component if needed.
Permanent magnets, in contrast to the widely used electromagnets that are currently in use at NSLS-II, would bring several benefits, particularly in their size and efficiency, but they also present some interesting challenges, namely the design and fabrication of these unique magnet modules.
Evolving prototypes
To test this revolutionary design, the accelerator team began developing prototypes at multiple scales. The initial prototype — a small, low-energy version — paved the way for a full-scale, high-energy model designed in collaboration with the Lab’s Collider-Accelerator Department. The next step is to install two full-scale prototypes in the NSLS-II accelerator tunnel to demonstrate their operational feasibility, a major milestone that calls for careful planning and cautious optimism.
“We are planning for success,” said Bernard Kosciuk, a mechanical engineer in the Accelerator Division’s Instrumentation Group. “Before installation, we need to take rigorous magnetic measurements in the lab that will instill a high level of confidence in the prototype’s performance. We want to address any uncertainty to ensure we can commission the prototype.”
These prototypes are a critical step toward developing an accelerator that uses the complex bend design, an approach that could deliver major benefits to NSLS-II. These permanent magnets will not require utility infrastructure or maintenance, and they will likely reduce power consumption and cost by an estimated 80%, from 1.7 megawatts to 0.3. The low emittance would increase brightness by an estimated factor of 10-100, depending on the photon energy, which would make experiments at the beamlines more efficient. The space saved by the compact size would leave ample room for insertion devices and other accelerator equipment, allowing for new possibilities at future beamlines.
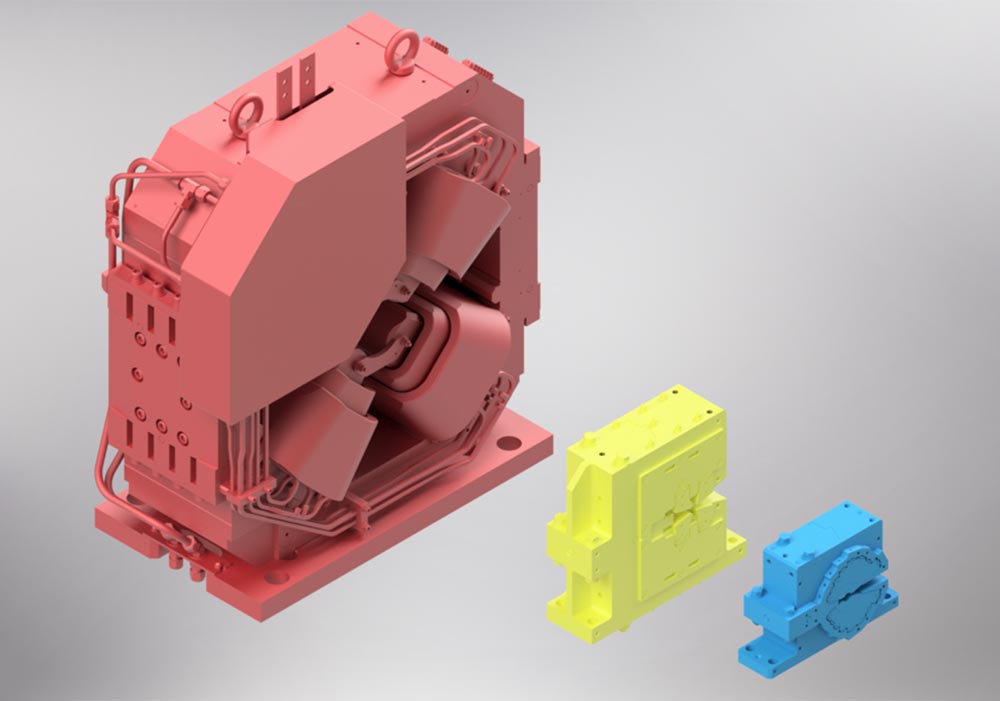
Compared to the National Synchrotron Light Source II's current quadrupoles (red), the permanent magnet quadrupoles (blue) and hybrid permanent magnet quadrupoles (yellow) designs are significantly more compact. (Richard Faussete/Brookhaven National Laboratory)
The six-year journey from concept to full-scale prototype has already built up momentum fairly quickly. Guimei Wang, leader of the accelerator coordination group at NSLS-II, led a team that successfully demonstrated the first complex bend prototype using the NSLS-II linac beam, a 200 megaelectron volt test setup, and a scaled-down version of a three gigaelectron volt machine. This first proof of concept would operate at 15 times less energy than a full-scale version.
“There were some challenges with this novel design,” recalled Wang. “Strong focusing made it difficult to match optics perfectly and avoid beam blowout. The compact size also made it difficult to incorporate diagnostic equipment. We were able to find innovative ways around some of these issues, including using machine learning to tune the beam, a flexible vacuum chamber connection, and a micrometer-resolution imaging system. The initial prototype ended up being a successful step in the project, though. The design maintained high gradient, comparable to a full-scale magnet, while significantly reducing the magnet size.”
This initial work led to a three-year Laboratory Directed Research and Development proposal to scale up the prototype to a single girder assembly with combined-function PMQs and a vacuum chamber. Vacuum systems are integral to accelerators like this, but the radically different magnet design would place significant challenges on the vacuum chamber.
“The incredibly small aperture and straight magnetic elements required precision machining to accommodate a smooth beam radius while also allowing sufficient exit slot geometry,” explained Robert Todd, leader of the Vacuum Group at NSLS-II “This pushed the limits of machining and welding to create a thin-walled chamber, two meters in length, within tolerance and capable of withstanding vacuum forces. A careful design and machining approach led to a successful vendor collaboration and fabrication.”
“We had to come up with creative solutions because nothing like this had really been done before,” said Sushil Sharma, a senior advisor in the Accelerator Division and the lead on this project. “To keep our facility competitive with the rest of the world, we have to do a lot of research and development ourselves.”
There was another aspect of this prototype that needed to be realized. The low energy version used simple PMQs that were able to focus and defocus, but bending was produced by displacing these magnets perpendicular to the beam. This new prototype needed to be designed to realize the multifunctional aspect of the magnets and allow bending to be produced by the PMQ itself at a larger size with optimized permanent magnet wedges, which required significant changes to their magnetization angles. These changes, which were crucial to move forward, were made possible through a collaboration with Brookhaven’s Collider-Accelerator Department. Stephen Brooks, an accelerator physicist within the department, was instrumental in developing the two-dimensional geometry for the PMQs.
Carefully closing in on the design of the future
An important aspect of the PMQs is their field quality, especially in combined function magnets. If a magnet is designed to have a dipole and quadrupole field, it should not show any other higher-order field. This was measured very precisely with a tiny copper coil that was created for the task in a collaboration with DOE’s Fermi National Accelerator Laboratory and ended up being a significant project in and of itself. Three of these coils were made and used to test the performance of this new magnetic measurement system. Passing those tests would lead to the biggest challenge yet.
“This prototype met the specifications we set, but to be installed in the storage ring, we need to validate its scalability, cost-effective production, and operational reliability,” said Sharma. “We’re currently working on the proposal to create two fully functional prototypes that fit these refined specifications and install them in the NSLS-II storage ring.”
These aren’t the only explorations that are happening. A team of scientists from NSLS-II’s Accelerator Division also participated in the recent “1,000 Scientist AI Jam Session” hosted by DOE in cooperation with OpenAI and Anthropic. At this event, teams of scientists and engineers from nine DOE national laboratories brought some of their toughest challenges to test advanced AI models and see how they could help with their research in the future.
“Our goal was to test the ability of AI tools to help us solve some of the problems related to the PMQ design in relation to the NSLS-II upgrade,” recalled Patrick N’Gotta, a magnet physicist in NSLS-II’s Accelerator Division who participated in the event. “We investigated the mathematical model of the magnet, ideas for field correction, ways to improve our correction algorithm code, and the possibility of predicting a correction based on previous correction data. Overall, we’re convinced that AI is an interesting tool that can help our work and save us time if provided with the proper input and guidance.”
As designs are being tested and data is being analyzed from each study, NSLS-II staff and members of the greater light source community have been putting their heads together in a number of discussions and workshops. In the last four years, the subject of an accelerator upgrade has been a topic of immense interest for scientists and engineers across the entire facility, with the complex bend being the core element for an upgraded machine. There is currently another large, exploratory science workshop with a focus on the accelerator upgrade being organized in early fall of 2025.
“Complex bend methodology conceived at NSLS-II is planned to become the main building block for a future facility upgrade, taking advantage of high-gradient, high-quality fields produced by complex permanent magnets for the low-emittance ring lattice design,” said Shaftan. “It will be really exciting to see the hard work that led up to where we are now become the accelerators of the future.”
Brookhaven National Laboratory is supported by the Office of Science of the U.S. Department of Energy. The Office of Science is the single largest supporter of basic research in the physical sciences in the United States and is working to address some of the most pressing challenges of our time. For more information, visit science.energy.gov.
Follow @BrookhavenLab on social media. Find us on Instagram, LinkedIn, X, and Facebook.
2025-22283 | INT/EXT | Newsroom