Introducing…sPHENIX!
A new collaboration takes aim at understanding how the ultra-hot, ultra-dense plasma that formed our early universe gets its intriguing properties.
June 15, 2016
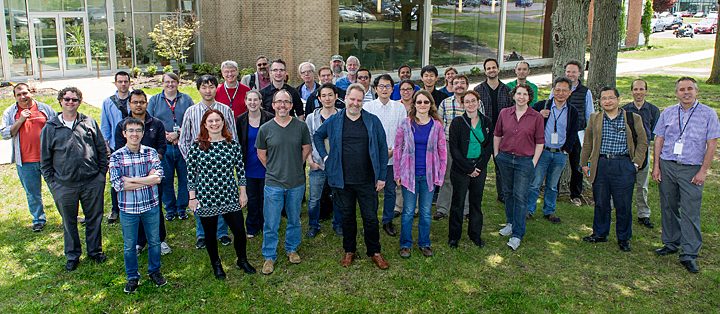
Members of the new sPHENIX collaboration at a meeting held at Brookhaven Lab in May 2016, with co-spokespersons Dave Morrison (green T-shirt, jeans) and Gunther Roland (blue shirt, black jeans) front and center.
From the very beginning, there were hints that particle collisions at the Relativistic Heavy Ion Collider (RHIC) were producing something unusual. This U.S. Department of Energy (DOE) Office of Science User Facility at Brookhaven National Laboratory was designed to recreate the incredibly hot and dense conditions of matter in the early universe by colliding atomic nuclei at high enough energies to “melt” their constituent protons and neutrons. The collisions would “free” those particles’ inner building blocks—quarks and gluons—so nuclear physicists could study their behavior unbound from ordinary matter.
Results from RHIC show that these particle smashups have indeed created a superhot primordial soup called “quark-gluon plasma” (QGP)—but one in which the quarks and gluons, though liberated from their protons and neutrons, continue to interact strongly. These strong interactions make the plasma flow like a nearly “perfect” liquid.
RHIC’s discovery of the perfect liquid set off a decade-long and very successful effort to characterize its remarkable properties—both at RHIC and at Europe’s Large Hadron Collider (LHC), where physicists conduct complementary studies of quark-gluon plasma for a few weeks of each year. But understanding exactly how the QGP’s perfect fluidity and other collective properties emerge from its point-like constituent particles remains a compelling mystery.
This is a new collaboration, and, if we get the go-ahead for this upgrade, this detector will have brand new capabilities.
— Brookhaven Lab physicist and sPHENIX co-spokesperson Dave Morrison
To address that mystery, a group of nuclear physicists has formed a new scientific collaboration that will expand on discoveries made by RHIC’s existing STAR and PHENIX research groups. This new collaboration, made up of veterans of the field and researchers just beginning their careers, has precise ideas about the measurements its members would like to make—and hopes of upgrading the PHENIX detector to make those measurements at RHIC.
“What remains to be done is to understand how the QGP’s properties arise or emerge from the underlying quark and gluon interactions,” said Massachusetts Institute of Technology physicist Gunther Roland, a longtime RHIC and LHC collaborator and now a co-spokesperson for the new collaboration..
Brookhaven physicist Dave Morrison, the other co-spokesperson, agrees: “On the one hand we have a very successful theory that describes the quarks and gluons as free point-like particles. On the other hand, we have a whole set of measurements that describe the collective properties of the QGP. What we’d like to do is connect the two—the microscopic to the not-so-microscopic.”
For now, the collaboration goes by the name sPHENIX: “s” for its focus on the strongly interacting particles and PHENIX for the anticipated use of key detector components and that experiment’s location in the RHIC ring once the existing PHENIX systems complete their data-taking lifetime at the end of this year’s run. But the collaboration leaders emphasize that there’s no need for members to be previously affiliated with PHENIX—or indeed with prior research at RHIC.
“This is a new collaboration, and, if we get the go-ahead for this upgrade, this detector will have brand new capabilities,” Morrison said.
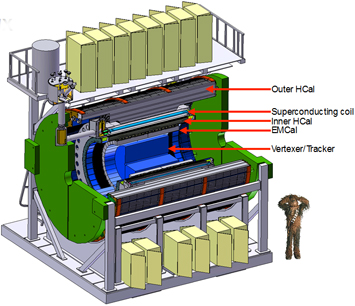
A schematic of the proposed sPHENIX detector, showing several key components: outer and inner hadronic calorimeters (HCal), electromagnetic (EM) calorimeter, tracking systems, and coils of the superconducting solenoid magnet
Tracking probes from within the plasma
Figuring out how the QGP’s properties emerge from its smallest particles requires a detector that can make more—and more precise—measurements of what’s going on in the plasma at different length scales.
“Think about looking at a pond that behaves like a liquid,” Morrison explained. “You might see waves and flowing water. If you had a microscope that could dial down, at some point you would see water molecules—the particles that make up the water. If you know a lot about those particles and how they behave, you can try to understand how the properties of the pond arise from the properties of the molecules. That’s what we’d like to do with the QGP.”
Particle detectors are the microscopes nuclear physicists use to dive down into the details of subatomic matter. But instead of shining visible light, electrons, or x-rays on the sample, particle detectors pick up signals from particles created within the collisions. Measuring how these particles move through and lose energy by interacting with the plasma will reveal information about the QGP at scales between the level of individual quarks and the long-scale collective behavior.
“There has to be an evolution from the short-wavelength behavior to the long-wavelength behavior, and we want to probe that transition,” Roland said.
Fast detector for precision measurements
One set of particles sPHENIX physicists are interested in tracking are upsilons—each made of two heavy quarks bound together. Each different bound state has a different mass. The sPHENIX scientists want to understand how upsilons with different masses form and disassociate and otherwise interact with the plasma.
They’re also interested in analyzing collimated streams of particles called jets—created as the energy of individual fast-moving quarks and gluons is transformed into a cascade of new particles. Measuring how much energy is lost by higher- and lower-energy jets will convey information about both the individual particle scale deep within the plasma and its long-range characteristics.
“The higher the momentum, the more rarely it is produced. So you need a very fast detector that can capture a lot of collisions to increase the chances of spotting these important events,” Roland said.
By removing outdated components from PHENIX and replacing them with new, custom-designed systems, the sPHENIX collaboration would transform that experiment into a “new” state-of-the-art detector that can capture as many as 15,000 events per second—a significant increase over STAR’s current capture rate of 2,000 events per second, or PHENIX’s 5,000—with all the components needed to differentiate among the three mass states of upsilons and tease apart the full energy scale of jets.
“This transformed detector would be suited to record a huge fraction of what RHIC can produce,” Morrison said.
Testing essential detector components
Physicists and engineers at Brookhaven and elsewhere have already begun building prototypes and testing components that could be used to achieve the anticipated transformation. And this endeavor is attracting a new generation of physicists eager to get in on the ground floor of a new experiment.
“I worked on PHENIX as grad student at Stony Brook University. Then, as a postdoc at Yale, I worked on the ALICE experiment at the LHC,” said Megan Connors, a RIKEN-BNL Research Center Fellow at Brookhaven Lab who will begin teaching and forming her own research group at Georgia State University next year. “When I came on the scene, both colliders were already up and running. So this is a chance to be involved from the start—to see how these experiments come to life, to be part of the formation of the collaboration and get involved in building the hardware in addition to analyzing the data.”
The piece of hardware that currently has her attention is a prototype “calorimeter” that would track and reconstruct the sprays of particles that make up jets, which recently underwent extensive testing at Fermi National Accelerator Laboratory.
“A typical jet may contain 10 or 15 particles, but you need to tease those out from the hundreds of particles coming out of a heavy ion collision event,” Connors said. “And you need to capture all the particles to be able to reconstruct the jet and see how much energy it loses as it travels through the plasma.”
You also need to know how much energy the jet had to start with. Most of the time jets are formed in back-to-back pairs. Both jets lose energy in the plasma. However sometimes, instead, a particle of light called a photon gets produced back-to-back with a jet. But unlike the jet particles, the photon shooting off in the opposite direction does not interact with the quarks and gluons in the plasma, so it doesn’t lose any energy.
“If you have a photon going one way, and a jet going the other way, the jet and the photon had the same starting energy,” explained Anne Sickles, an sPHENIX collaborator from the University of Illinois at Urbana-Champaign who was also involved in the calorimeter design and testing. “So measuring the photon’s energy gives you the starting point. Measuring the particles that make up the jet and subtracting from the photon energy tells you how much energy the jet lost.”
Using Fermilab’s Test Beam Facility, Sickles and some of her students shot a beam of electrons through portions of an “electromagnetic” calorimeter they designed to track photons and some of the other particles that make up jets. For the initial tests, the electrons—pure electromagnetic particles like photons—served as stand-ins for the photons. The aim of the tests was to be sure all areas of the detector respond in a similar way, and that there’s no variation between pieces built by Sickles and her students in Illinois and pieces constructed by an outside contractor.
Next, the physicists added components of a “hadronic” calorimeter for tracking hadrons (particles made of more than one quark), which Connors and her team had been working on. They placed the hadron detectors directly behind the electromagnetic calorimeter—just as the two components will be arranged in the actual detector. This outer layer is designed to catch the larger hadron particles that make it through the first layer so physicists can account for the full energy of each jet.
Building the calorimeter thick enough to “catch” all the particles is one way that the design of sPHENIX benefits from the 16 years of operating RHIC and several years experience at LHC.
“Before RHIC was built, we didn’t even know how many particles would be produced. We had to build the detectors to cover a wide range of possibilities,” Morrison said. “Now, knowing what the collisions look like and the kinds of particles produced, we can build a detector tailored to do the measurements that are focused on the specific important questions we’d like to answer.”
Mighty magnet
Testing is also underway on a 20-ton solenoid magnet acquired from a former physics experiment at DOE’s SLAC National Accelerator Laboratory. This magnet would form the heart of the sPHENIX detector, completely surrounding the collision zone like the cylindrical magnet at the center of RHIC’s STAR detector. Like STAR’s, the sPHENIX magnet would bend the trajectories of charged particles as they emerge from the collisions. But with three times the bending power of STAR, sPHENIX should be able to separate out the signals from the three types of upsilon particles, whose masses differ by only a few percent.
“Upsilons don’t make it all the way to the magnet,” Morrison explained. “These are heavy particles that decay, often into an electron and an antielectron, which have a lot of energy when they come out. You need a powerful magnetic field to bend these charged particles so you can get a better measurement of their velocity and momentum, and tease out small differences to separate the electrons that come from the different-size upsilons.”
So far, a team of engineers and physicists in Brookhaven’s Superconducting Magnet Division, Collider-Accelerator Department, and Physics Department has cooled the superconducting magnet down to its near-absolute-zero operating temperature of 4.2 Kelvin and tested it with 100 amperes of current.
“We needed to test the overall health and integrity of the magnet to make sure all the joints and couplings are in place, in case they got jostled while being transported cross-country,” said lead magnet engineer Piyush Joshi. They also tested systems Joshi designed to shut the magnet down in a controlled manner if the field between the magnet’s two layers of coils ever gets out of balance. “You want to detect any imbalance very quickly so you can extract the energy before it causes any damage to the magnet,” he said. He originally wrote the algorithms for an LHC magnet project, but they proved to be just as useful for the sPHENIX tests.
With the initial, low-field tests complete, the group will next use steel recycled from another older experiment at Brookhaven to surround the magnet to contain its most powerful field—and ramp it up to a full 4,600 amps.
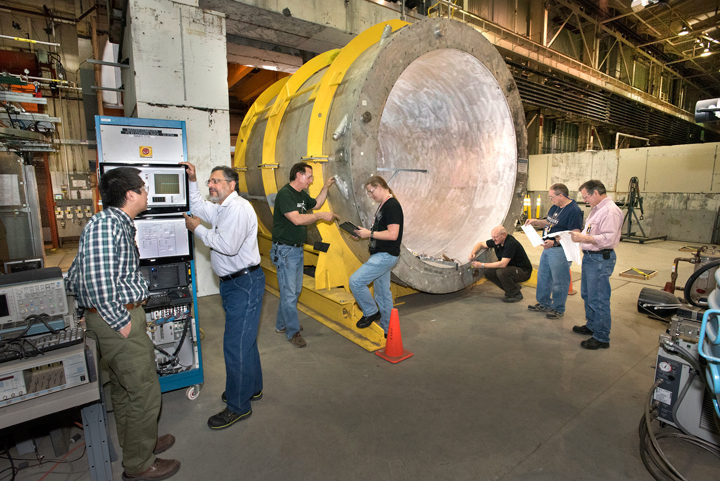
Engineers and physicists involved in testing the 20-ton superconducting solenoid expected to form the heart of the sPHENIX upgrade: Kin Yip, Collider-Accelerator Department (CAD); Piyush Joshi, Superconducting Magnet Division (SMD); Richard Meier, CAD cryo group; Brian Van Kuik, CAD main control room operations coordinator; Ray Ceruti, SMD; Sonny Dimaiuta, SMD; Dominick Milidantri, SMD.
Path forward
By reusing equipment and tools developed with funding for RHIC and the LHC, and inspiring university collaborators to chip in their expertise, the nascent collaboration has taken these early steps on the path toward transforming PHENIX into sPHENIX. But the team hopes to get an official seal of approval—and, eventually, a budget—from DOE.
The 2015 Long Range Plan for Nuclear Science—a set of recommendations made by the nation’s Nuclear Science Advisory Committee to leaders at DOE and the National Science Foundation—identifies the sPHENIX “state-of-the-art jet detector” as “essential” to probing the inner workings of QGP at shorter and shorter length scales, one of two “central goals” noted in the report for completing the scientific mission at RHIC. The report also notes that there is significant international interest in sPHENIX.
“Right now we have a collaboration of 183 people, and growing,” Morrison said, with those scientists representing 58 institutions in 10 countries.
Looking ahead and continuing the tradition of making the most of our nation’s investments in science, the physicists designing the sPHENIX upgrades say this transformed detector could largely be reused as a detector for a future Electron Ion Collider—the next priority nuclear physics project identified in the Long Range Plan.
“Transforming PHENIX into sPHENIX would maximize the benefits derived from the investments already made to build RHIC by allowing us to fully understand the quark-gluon plasma,” Morrison said. “It’s what we need to do to complete the story of QGP discovery and to prepare for the coming research directions in nuclear physics.”
sPHENIX R&D is supported by the DOE Office of Science and also by Brookhaven Lab’s Laboratory Directed Research and Development program, BNL Program Development, and in-kind contributions from collaborating universities.
Brookhaven National Laboratory is supported by the Office of Science of the U.S. Department of Energy. The Office of Science is the single largest supporter of basic research in the physical sciences in the United States, and is working to address some of the most pressing challenges of our time. For more information, please visit science.energy.gov.
2016-6258 | INT/EXT | Newsroom