- Home
-
Research Groups
Division Groups
- Artificial Photosynthesis
- Catalysis: Reactivity & Structure
- Electrochemical Energy Storage
- Electron- and Photo-Induced Processes for Molecular Energy Conversion
- Neutrino and Nuclear Chemistry
- Surface Electrochemistry and Electrocatalysis
Associated Groups
- Catalysis for Alternative Fuels Production
- Nanostructured Interfaces for Catalysis
- Structure and Dynamics of Applied Nanomaterials
- People
- Operations
- News
- Events

Catalysis: Reactivity and Structure
The Catalysis Group has research efforts in the areas of:
- Fundamental studies in C1 Chemistry: CO2 conversion to alcohols and methane, water-gas shift reaction, activation and conversion of methane.
- Development of techniques for in-situ characterization of model and powder catalysts.
- Theoretical modelling of well-defined model/powder catalysts and catalytic chemistry.
- Studies of the catalytic properties of metal-oxide, metal-carbide and metal-sulfide interfaces.
- Metal-support interactions and the tuning of the catalytic activity of noble and late transition metals.
- Synthesis and characterization of model catalysts with an inverse configuration.
- Transient studies linking reaction mechanism and catalyst conversion.
A brief description of these efforts is summarized below.
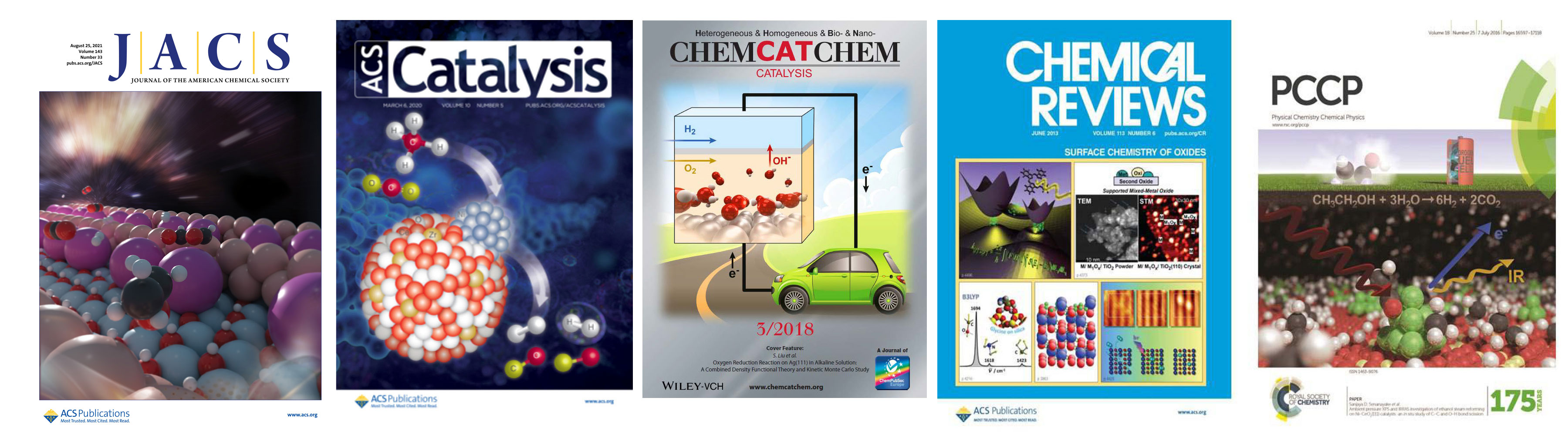
Development of Techniques for In-situ Characterization of Powder Catalysts
Time-resolved PDF, XAFS/XRD, XAFS/IR and XAFS/Raman
(Rodriguez, Senanayake, Hanson)
The development of techniques for characterizing the structural properties of catalysts under the high-pressure conditions of industrial processes is widely recognized as a top priority in the area of heterogeneous catalysis. In the last several years, the CRS Group at BNL has been very active in developing instrumentation for the characterization of catalysts with pair-distribution function (PDF) analysis and the integration of XAFS and XRD or XAFS and IR or Raman.
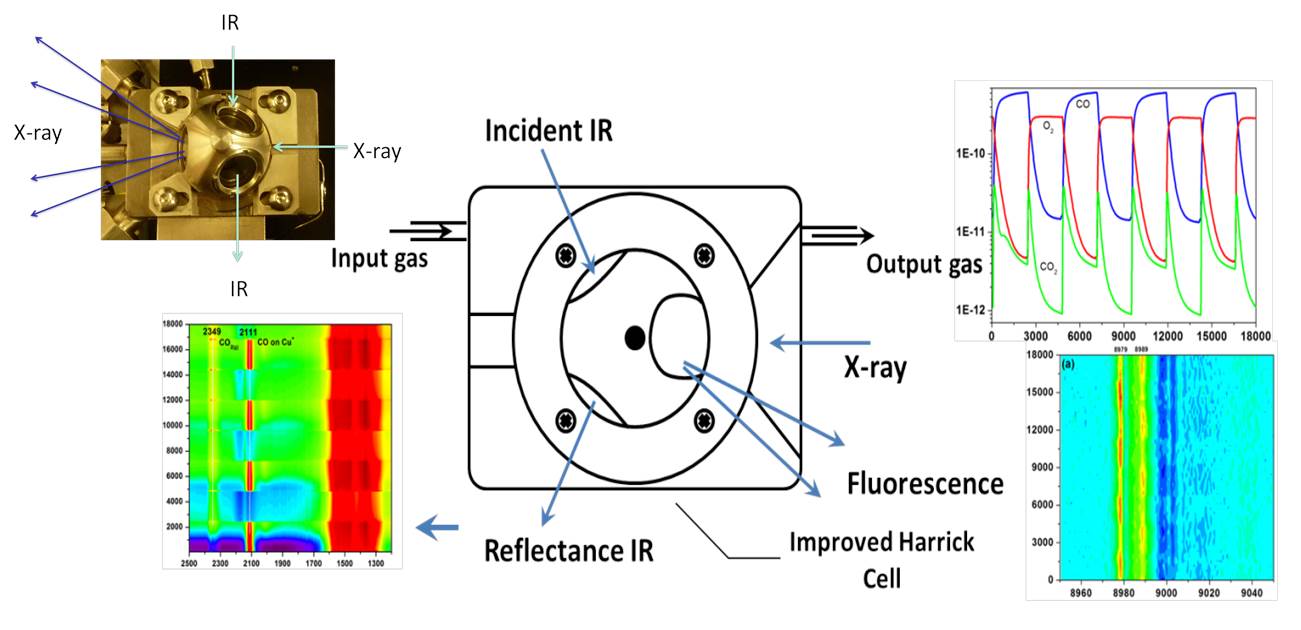
Schematic of the set-up for combined, operando XAS/DRFTS experiments.
The integration of IR and Raman with XAFS allows a simultaneous determination of the reaction intermediates on the surface and the chemical state and structure of the catalyst. Raman/DRIFTS spectroscopy adds sensitivity to molecular surface information that both XANES and EXAFS are lacking. In-situ PDF has been used to study the structure of amorphous metal and metal oxide nanoparticles. Using a XAFS/IR combination, we have investigated reaction mechanisms and correlations between structure/reactivity for CO oxidation and ethanol steam reforming on Cu-ceria and Ni-ceria catalysts, and a combination of XAFS/Raman was used to study the oxidation of CO on a Cu/TiOx catalysts. The Catalysis Group has been strongly involved in the design and planning of new beamlines at NSLS-II including for XRD/(IR or Raman), PDF/(IR or Raman) at XPD; XAFS/(IR or Raman) at ISS, ambient-pressure XAS, and ambient-pressure XPS at CSX-2. These beamlines are in operation and give the Catalysis Group a unique set of tools to study in-situ the structure of catalyst and reaction mechanisms.
Theoretical Modelling of well-defined model/powder catalysts and catalytic chemistry
Within the integrated approach to catalysis, theoretical and experimental studies are designed to strongly interact with each other via various ways. To gain better understanding of the experimental observations, combined DFT calculations and kinetic modeling is carried out to describe the complex catalytic structures that can be observed experimentally (e.g. STM), predict the major intermediates that can be measured experimentally (e.g. TPD or IR), and estimate the relative yield and selectivity under reaction conditions that can be compared with experimental data from batch/flow reactors. In this way, meaningful insights to the active sites, the optimal reaction pathway and the underlying mechanism for the catalytic behaviors observed experimentally can be provided.
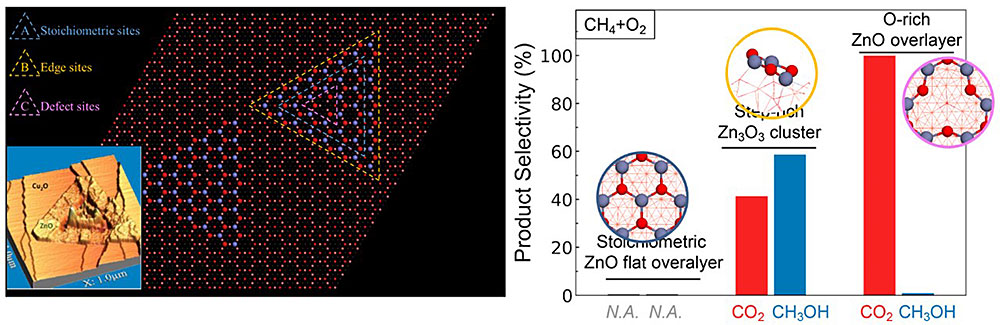
Data-driven descriptor identification, mechanic understanding and catalyst optimization.”
Data-driven machine learning models are developed to identify the effective descriptors that control the activity and selectivity of a catalyst based on the DFT-based kinetic modeling. The identified descriptors can be used for the accurate and efficient prediction of catalytic behaviors, and thus the optimization of advanced catalysts. By unraveling the role of each effective descriptor using model-agnostic interpretation method, the mechanic understanding of reaction kinetics can be also enhanced.
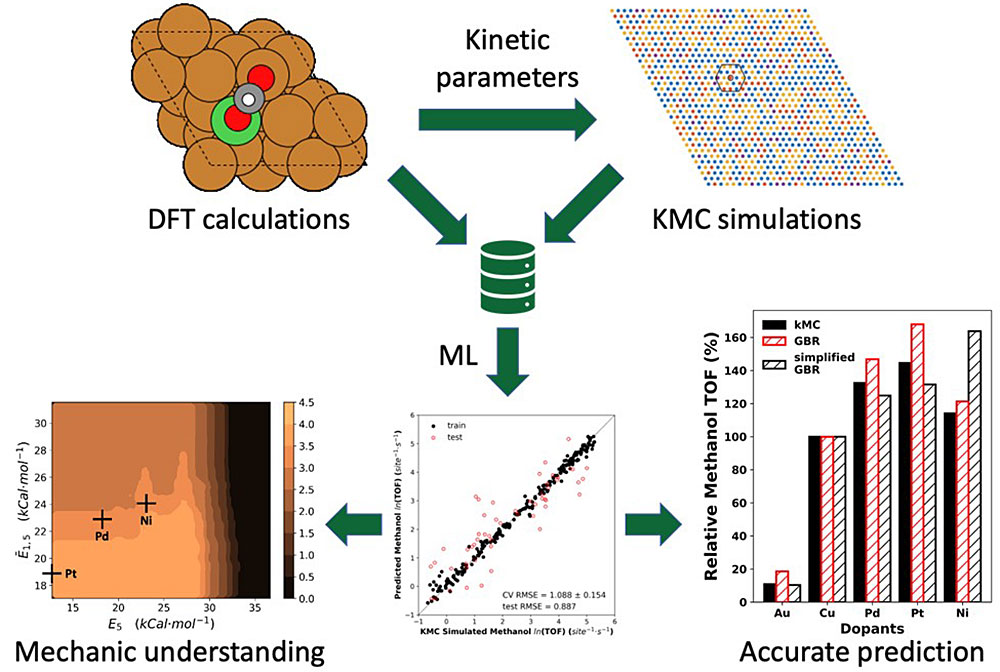
Well-defined Surfaces of Mixed-Metal Oxides
(Liu, Rodriguez, Senanayake)
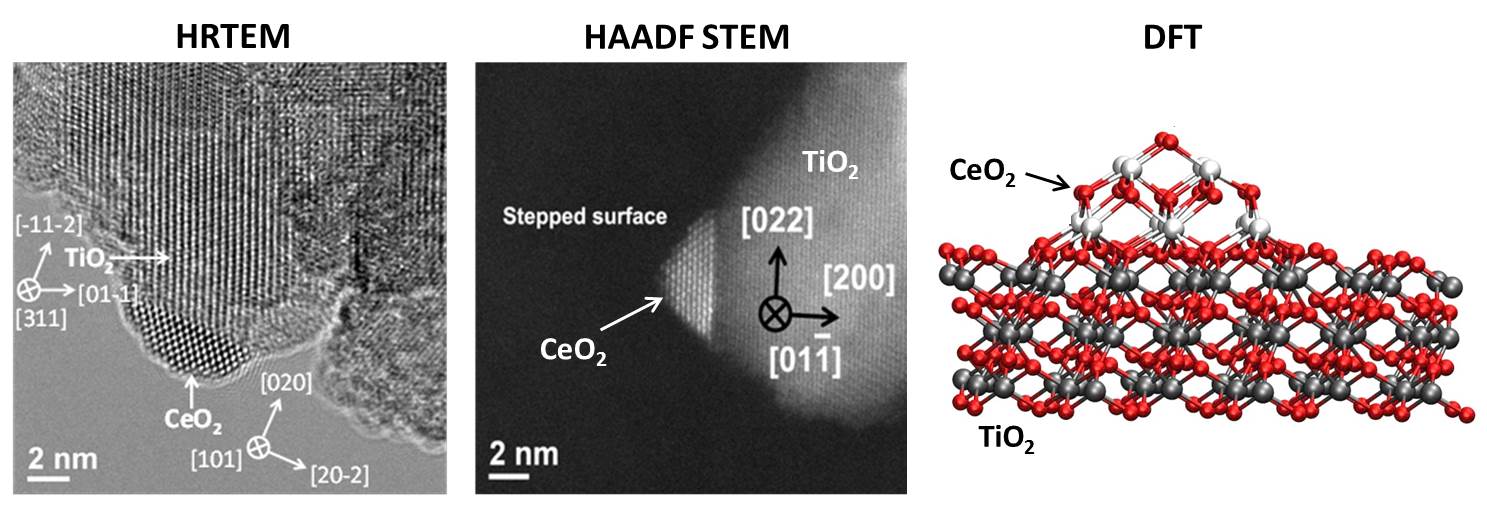
CeOx-TiOx mixed-metal oxide catalysts imaged using HRTEM and STEM. DFT model of CeOx-TiOx.
Our group has synthesized atomically resolved mixed-metal oxides of CeO2/TiO2, CeO2/CuOx , RuO2/TiO2 and others. Their catalytic properties have been tested over several reactions with and without the presence of low loading supported metals. A rich heterogeneity exists at the interface between two oxides and upon addition of metals provides a powerful combination of active sites that have proven to be highly active in activating C1 reactions. The true nature of the active sites in mixed metal oxides is sensitive to the morphology, electronic and chemical nature of the oxide nanostructures and yields.
Well-defined Surfaces of Inverse Catalysts
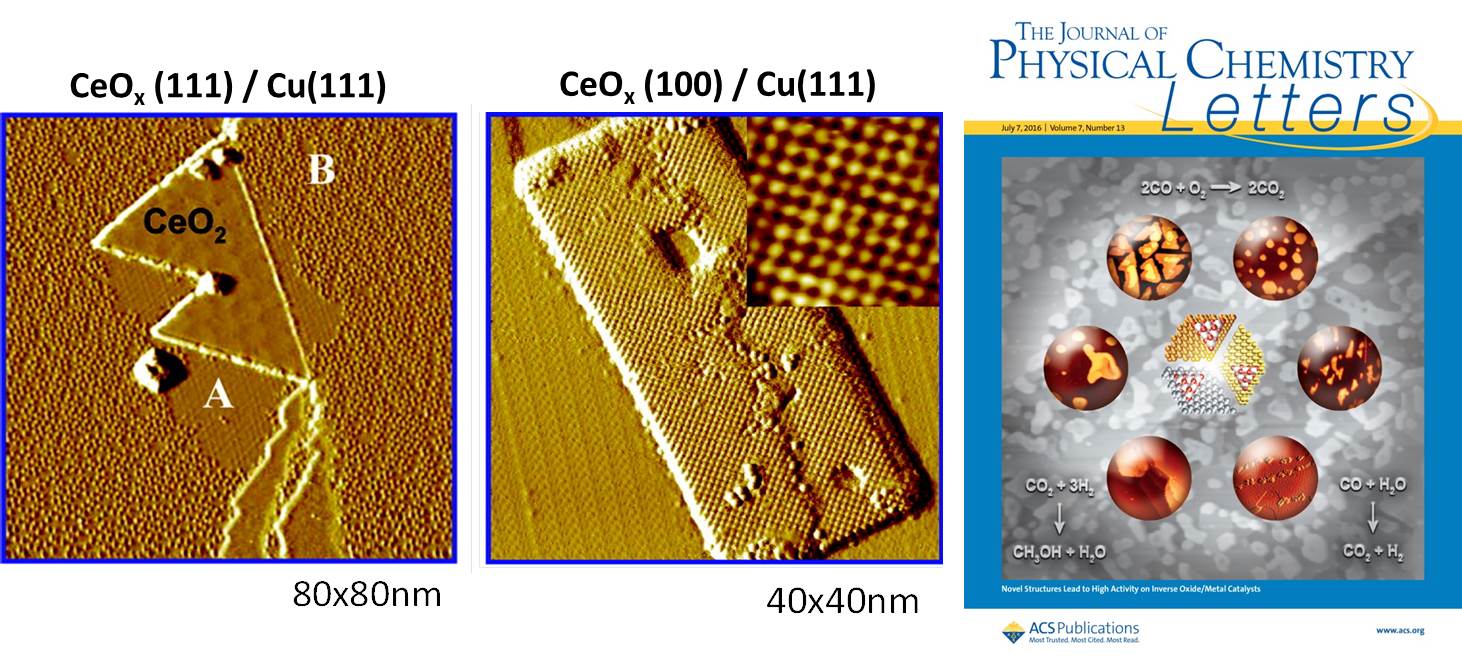
CeOx-Cu(111) inverse catalysts imaged using STM.
Inverse oxide/metal catalysts have shown to be excellent systems for studying the role of the oxide and oxide–metal interface in catalytic reactions. These systems can have special structural and catalytic properties due to strong oxide–metal interactions difficult to attain when depositing a metal on a regular oxide support. Oxide phases that are not seen or are metastable in a bulk oxide can become stable in an oxide/metal system opening the possibility for new chemical properties. Using these systems, it has been possible to explore fundamental properties of the metal–oxide interface (composition, structure, electronic state), which determine catalytic performance in the oxidation of CO, the water–gas shift and the hydrogenation of CO2 to methanol. Recently, there has been a significant advance in the preparation of oxide/metal catalysts for technical or industrial applications. One goal is to identify methods able to control in a precise way the size of the deposited oxide particles and their structure on the metal substrate. Recently, progress has been made for the preparation of inverse In2O3/Au(111) and ZrO2/Cu(111) catalysts for the selective conversion of CO2 to methanol.
Activation of Noble Metals on Metal-Carbide Surfaces: Novel Catalysts for CO2 Hydrogenation and Desulfurization Reactions
Rodriguez, Liu
In the continuous search for new catalysts, one may wonder whether metals deposited on supports other than metal oxides may provide alternative catalysts with increased activity or selectivity. Transition-metal carbides exhibit broad and amazing physical and chemical properties. High-resolution photoemission, scanning tunneling microscopy (STM) and first-principles periodic density-functional (DF) calculations have been used to study the interaction of metals of Groups 9, 10 and 11 with MC(001) (M= Ti, Zr, V, Mo) surfaces. DF calculations give adsorption energies that range from 2 eV (Cu, Ag, Au) to 6 eV (Co, Rh, Ir). STM images show that Au, Cu, Ni and Pt grow on the carbide substrates forming two-dimensional islands at very low coverage, and three-dimensional islands at medium and large coverage.
In many systems, the results of DF calculations point to the preferential formation of admetal-C bonds with significant electronic perturbations in the admetal, enhancing the chemical reactivity of the catalyst. Among all greenhouse gases in the atmosphere, carbon dioxide plays a special role due to the magnitude of the emissions generated by human activities involving the combustion of carbonaceous fuels, principally wood, coal, oil and natural gas. The recovery of CO2 for its hydrogenation to alcohols or other hydrocarbon compounds is an important approach to recycle the released carbon dioxide. This is a difficult task in C1 chemistry due to the challenges associated with the chemical activation of CO2. Can metals deposited on carbide supports be active enough for methanol synthesis? Our studies indicate that small Cu and Au particles in contact with a TiC(001) surface undergo a charge polarization which makes them very active for CO2 activation and the catalytic synthesis of methanol. They are also active as low temperature water-gas shift catalysts.
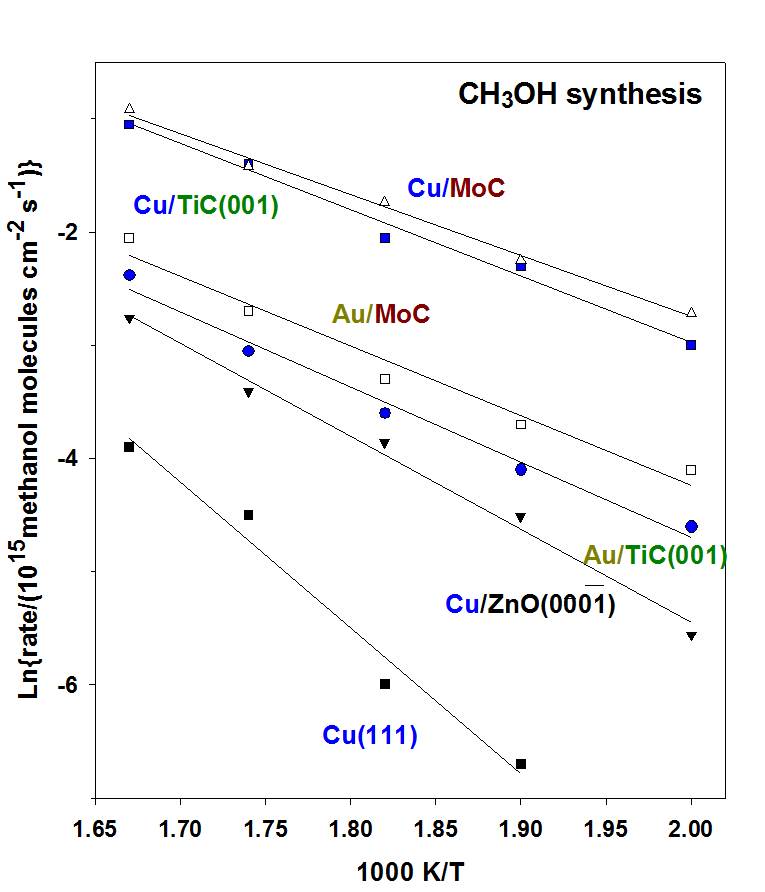
Arrhenius plot for the formation of methanol from CO2 hydrogenation on a series ofAu/carbide and Cu/carbide catalysts. PH2= 4.5 atm; PCO2= 0.5 atm.
Synthesis and Reforming of Alcohols on metal-oxide interfaces
a). Synthesis of methanol on ZnO/Cu(111), CeOx/Cu(111) and Cu/CeOx/TiO2(111) catalyst
Cu(111) has a low activity for the formation of methanol from CO2 hydrogenation. The deposition of ZnO or CeOx nanoparticles on Cu(111) produces an enhancement of 3-4 orders of magnitude in the rate of CO2 methanol synthesis. The formation of ZnO-copper and ceria-copper interfaces opens the possibility for new pathway for the adsorption and activation of the CO2 molecule not seen on pure metals. The bound CO2 undergoes hydrogenation to form key HOCO and HCOO intermediates that eventually lead to CO and methanol formation. The results of AP-XPS and FT-IRAS point to this type of chemistry on CeOx/Cu(111) and ZnO/Cu(111) surfaces. An even better catalyst is found after coadsorbing nanoparticles of Cu and CeOx on TiO2.
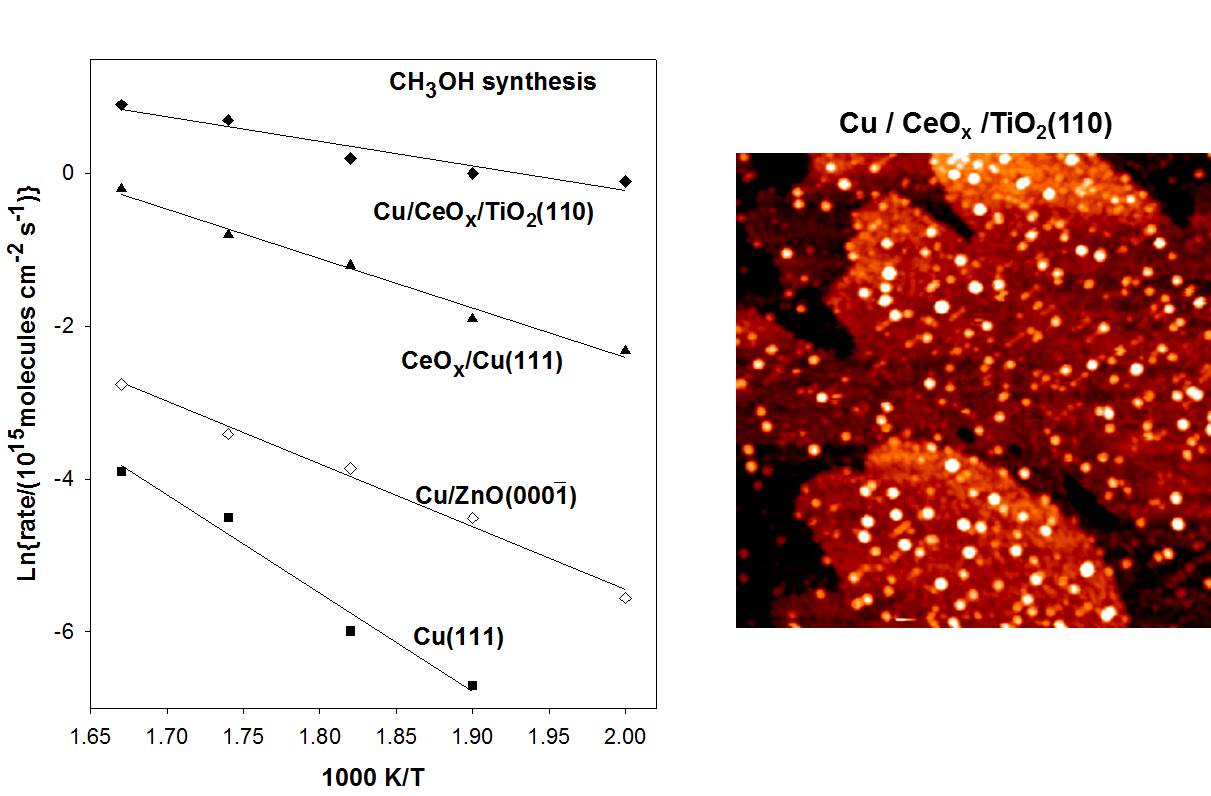
Arrhenius plot for the formation of methanol from CO2 hydrogenation on a series of Cu-oxide catalysts. PH2= 4.5 atm; PCO2= 0.5 atm.
b). Studies on Ethanol Steam Reforming on a Ni/CeO2 Catalyst
(Liu, Rodriguez, Senanayake)
In recent years, ethanol has received significant attention as a source of energy because it can be easily obtained from renewable energy sources. One objective is the production of hydrogen from steam reforming (C2H5OH + 3H2O → 2CO2 + 6H2). A major issue is the identification of non-expensive catalysts which can accomplish the cleavage of the C-C bond without subsequent deactivation by coke deposition. Our studies have shown that the NiO/CeO2 can accomplish this. The steam reforming of ethanol on a Ni-based CeO2-supported catalyst, was studied using in-situ X-ray XRD, operando DRIFTS, and mass spectroscopy with a focus on the structural characterization of the catalysts, chemical identification of the reaction pathway, and understanding of the interaction between Ni and CeO2.
We observed that the Ni electronic structure is modified by its interaction with the ceria support, modifying its catalytic properties. In the future, we plan to use this phenomenon defined as Electronic Metal-Support Interactions (EMSI) to tune the activity of metal/oxide catalysts.
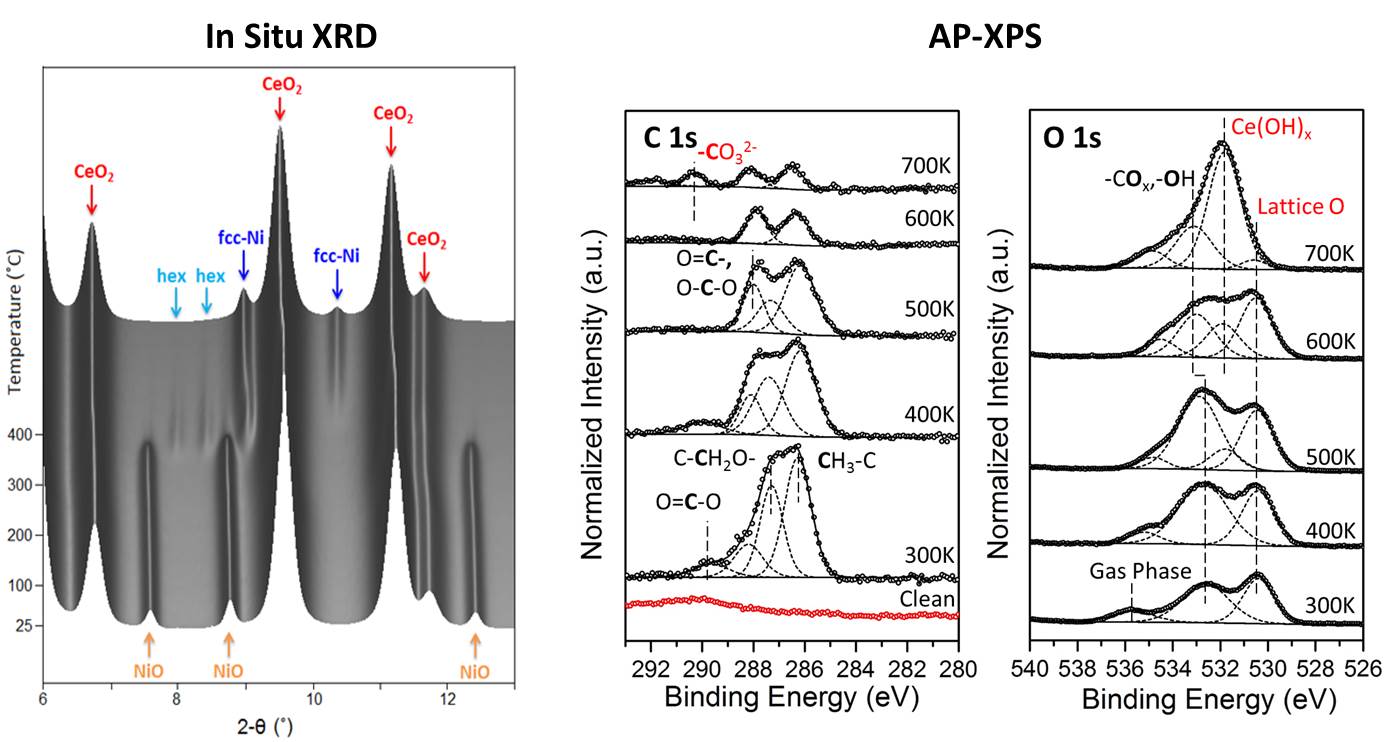
In situ XRD (left) and AP-XPS (right) data of Ni/CeO2 powder and model catalysts, respectively under ESR reaction conditions.
c). Methane activation – Synthesis of Alcohols and Dry Reforming
(Liu, Rodriguez, Senanayake)
Conversion of methane is a challenging process with few pathways that can upgrade methane directly in a single step. Ni-CeO2 is a highly efficient, stable and non-expensive catalyst for methane dry reforming at relative low temperatures (700 K). The active phase of the catalyst consists of small nanoparticles of nickel dispersed on partially reduced ceria. Experiments of ambient pressure XPS indicate that methane dissociates on Ni/CeO2 at temperatures as low as 300 K, generating CHx and COx species on the surface of the catalyst. Strong metal–support interactions activate Ni for the dissociation of methane. The results of density-functional calculations show a drop in the effective barrier for methane activation from 0.9 eV on Ni(111) to only 0.15 eV on Ni/CeO2−x(111). At 700 K, under methane dry reforming conditions, no signals for adsorbed CHx or C species are detected in the C 1s XPS region. The reforming of methane proceeds in a clean and efficient way.
Alternatively, an inverse CeO2/Cu2O/Cu(111)
catalyst is able to activate methane at room temperature producing C, CHx
fragments and COx species on the oxide surface. The addition
of water to the system leads to a drastic change in the selectivity of
methane activation yielding only adsorbed CHx fragments. At a
temperature of 450 K, in the presence of water, a CH4 → CH3OH
catalytic transformation occurs with a high selectivity. OH groups
formed by the dissociation of water saturate the catalyst surface,
removing sites that could decompose CHx fragments, and
generating centers on which methane can directly interact to yield
methanol.
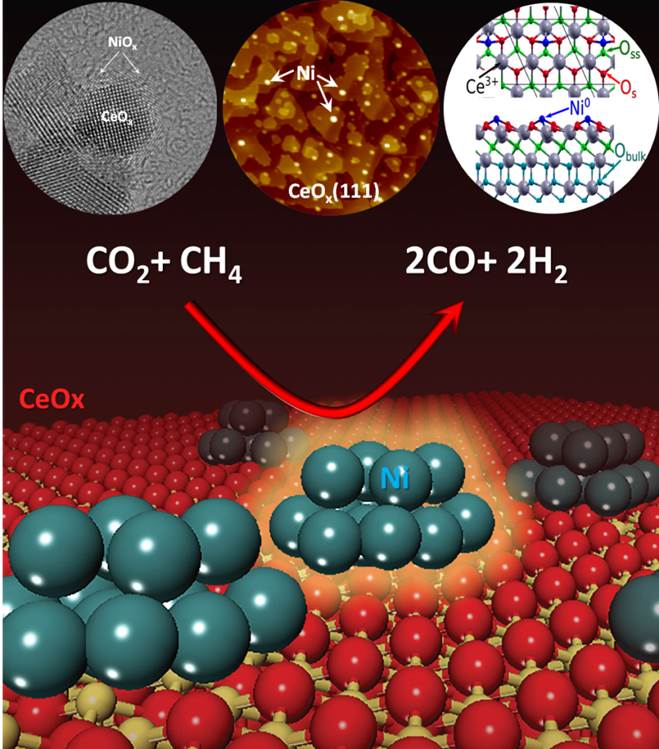
Dry Reforming of Methane studied using powder, model and DFT of Ni-CeOx