Nikhil Tiwale: Practicing the Art of Nanofabrication
As a postdoctoral researcher in the Center for Functional Nanomaterials at Brookhaven Lab, Tiwale fabricates new kinds of microelectronic device components
December 21, 2020
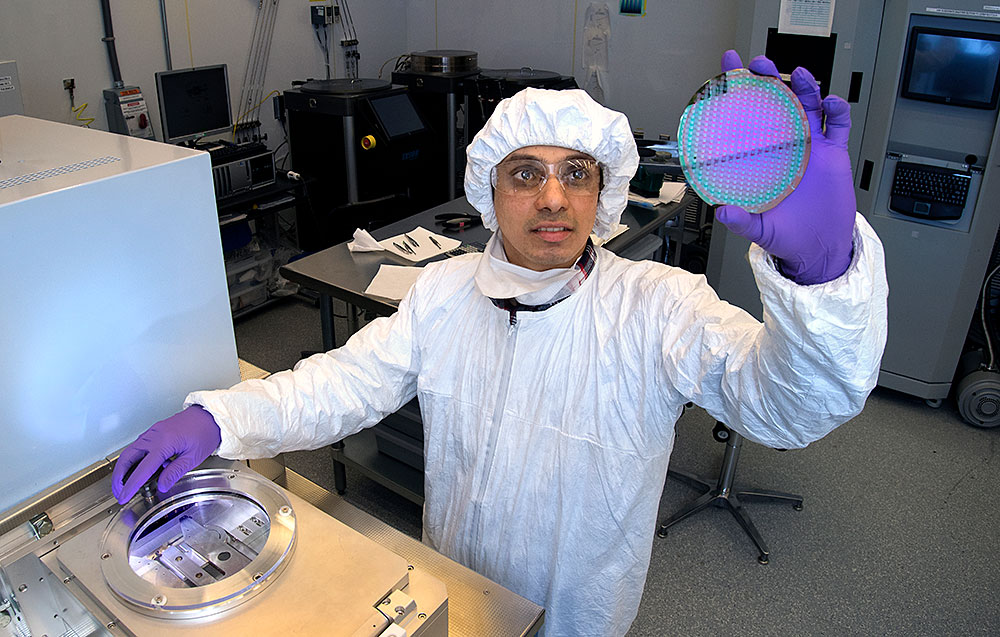
Nikhil Tiwale holds a nanopatterned silicon wafer in the Nanofabrication Facility cleanroom of Brookhaven Lab's Center for Functional Nanomaterials (CFN). Here, he processes resists (materials that are sensitive to external stimuli such as light, electrons), patterns them using lithography, and transfers lithographic patterns onto substrates like silicon through etching to build functional electronic devices.
From a young age, Nikhil Tiwale was curious about how technologies—particularly computers—are put together to deliver certain functions. At the same time, Tiwale found himself drawn to graphic art, fascinated with how the convergence of geometric shapes gives rise to intricate designs. He would spend hours sketching and shading with pencils and crayons.
As he grew up and delved deeper into how computers are made, he became intrigued by the materials that have enabled the integration of electronic devices into computer processors. So, when it came time to select his undergraduate major, Tiwale ultimately chose materials science and engineering. He was accepted to his dream school, the Indian Institute of Technology (IIT) Bombay, one of the top engineering universities in India.
“Computers initially used vacuum tubes as electronic switches and took up whole rooms,” said Tiwale. “Now, we have million-times-faster computers that we can carry in our pockets. To a large extent, advances in materials science made storing and processing information on tiny chips possible. This dramatic improvement in performance is one of the main reasons that I wanted to study materials science and engineering in college.”
But his artistic side never left him. Students at IIT Bombay were encouraged to participate in extracurricular activities, and Tiwale decided he would hone his art skills. He taught himself how to use advanced graphic design software like Photoshop, and graphic design became one of his hobbies. Little did he know that his artistic foundations would prove to be instrumental in his scientific career.
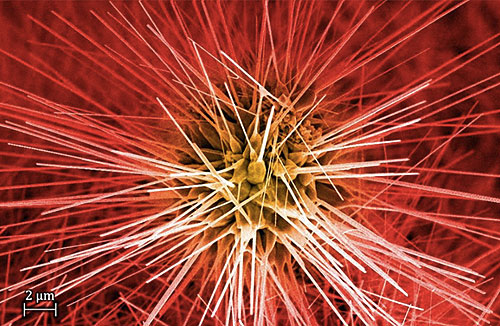
A nanowire "firecracker." The scanning electron microscope image is of zinc oxide nanowires that Tiwale and his PhD colleague grew on a graphite flake via thermal chemical vapor deposition. The University of Cambridge's Engineering Department publicized this image in "The art of engineering: images from the frontiers of technology" annual photography competition in 2014.
Tiwale graduated from IIT Bombay with a bachelor’s degree in metallurgical engineering and materials science, and a master’s in ceramics and composites. Then, he pursued a PhD in solid-state electronics and nanoscale science at the University of Cambridge in the United Kingdom. For his PhD research, which was under the guidance of Sir Mark Welland, Tiwale sought to develop a scalable method for making devices from zinc oxide nanowires. These one-dimensional wire-shaped structures have a diameter smaller than 100 nanometers, roughly the size of a virus. When charges are confined to a single dimension, unique electronic and optical properties emerge.
“At the time, most of the research in the field of oxide nanostructures had been focused on growing high-quality nanowires on one substrate, sprinkling these nanowires on a device-compatible substrate, making devices one by one, and then trying to understand how the material is performing,” explained Tiwale. “But this process isn’t scalable for making complex circuits or computer chips, for example. The first thing that comes to my mind regarding scalability for any semiconductor device is lithography-based patterning.”
The art of lithography
A Greek word that translates to “writing on stones,” lithography is a technique for generating patterns on material surfaces (substrates) through exposure to light, electrons, ions, or other external stimuli. Lithography is the primary technique that has enabled the precision patterning of electronic device structures. For example, to make integrated circuits, a light-sensitive material called a photoresist is coated onto a thin wafer of silicon. The resist is then selectively exposed to light through a “mask” containing the geometric pattern for the required electronic circuit.
“Photolithography is like taking a photograph,” explained Tiwale. “You take a snapshot of an entire circuit diagram and simultaneously print that onto a substrate. On the other hand, electron-beam lithography (EBL) is like drawing or sketching. You pattern one structure at a time and combine these structures to make your final circuit. To mass produce devices, you need both—the sketching-like lithography to design and optimize the structures, and the photography-like lithography to simultaneously transfer these structures onto a substrate at a fast pace.”
Using unique zinc-based precursor (reactant) materials that are sensitive to electrons, Tiwale developed an EBL process for the direct patterning of zinc oxide nanowires at desired locations on device substrates.
“With this process, hundreds and thousands of nanowire devices can be made simultaneously, meaning you can design circuits and more complicated structures,” said Tiwale, who used the process to make transistors (the building blocks of computer circuits) and gas sensors capable of detecting and distinguishing different vapors.
For Tiwale, lithography is as much a science as it is an art.
“I think of lithography as architecture at the micro or nanoscale,” said Tiwale. “Making an integrated circuit or chip is like architecting a several-story building. The physical layout of the processor architecture is the “floorplan,” and each microprocessor contains tens of layers, or floors. To make these integrated structures, you not only need an understanding of materials science but also graphic design skills. The scientific and artistic sides come together.”
One of Tiwale’s major inspirations is Leonardo da Vinci, who had a passion for both art and science.
“da Vinci is famously known for his artistic masterpieces like the Mona Lisa, but he also drew detailed sketches of human anatomy and aircraft blueprints,” said Tiwale. “He imagined science and technology centuries before they were materialized.”
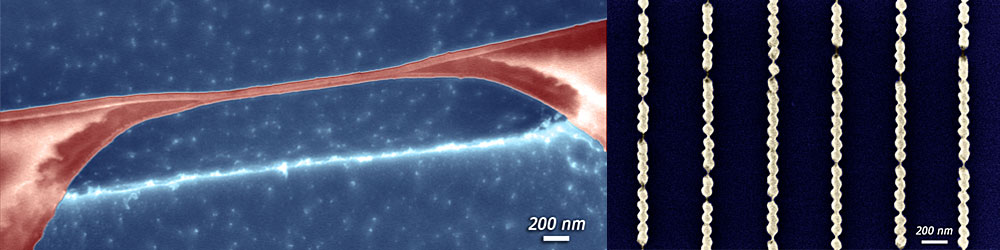
(Left) A scanning electron microscope image of a suspended nanostructure fabricated using electron-beam lithography (EBL) and reactive-ion etching. (Right) A series of polymeric nanodots patterned during a suboptimal EBL run. As Tiwale explained, "scientific experiments do not always lead to perfect outcomes, but they can depict beautiful structures nonetheless."
Aligned research themes
In 2017, toward the end of his PhD program at Cambridge, Tiwale began looking for postdoc job openings and came across a position describing a project very similar to the one he had been working on. The position was in the Electronic Nanomaterials Group of the Center for Functional Nanomaterials (CFN)—a U.S. Department of Energy (DOE) Office of Science User Facility at Brookhaven National Laboratory. In this group, CFN staff scientists Chang-Yong Nam and Aaron Stein were also using EBL to make polymer-based nanoscaffolds for oxide nanodevices. While Tiwale’s technique was limited to a single metallic precursor, Nam’s and Stein’s could be applied to different metals. Therefore, they could explore materials with a variety of properties beyond those ideal for transistors and gas sensors.
“The position had a good overlap with my PhD research, and I’d have the chance to extend the research to different materials and applications,” said Tiwale.
Tiwale was also attracted to the user-oriented nature of the CFN. At the University of Cambridge, he had conducted his PhD research in The Nanoscience Centre, a university-wide user facility. Here, Tiwale served as an instructor for new users of EBL and other cleanroom-based processing tools.
“I really liked how my regular interactions with members of various departments across the university pulled me out of my narrow research focus and got me thinking about broader ideas in science,” said Tiwale. “As a worldwide user center, CFN takes this interaction to another level.”
Postdoctoral research
Since March 2018, Tiwale has been a postdoc in the CFN Electronic Nanomaterials Group. Initially, he focused his research on infiltration synthesis, a technique for growing inorganic materials within polymers by introducing precursors in gas form. Working in the CFN Materials Synthesis and Characterization Facility, Tiwale used this technique to produce inorganic metal oxide nanostructures. One of the applications of interest to the group is the fabrication of metal oxide nanowires, like those Tiwale was making during his PhD studies, for functional applications.
Last year, Tiwale was the lead experimentalist on a team who used infiltration synthesis to make “hybrid” resists—those that combine organic polymer-based materials with inorganic materials like zinc, tin, and aluminum. As Tiwale explained, the microelectronics industry has been moving toward extreme-ultraviolet lithography, or EUVL, to further miniaturize device features. EUVL requires new resist materials that are sensitive to extreme ultraviolet light. The addition of inorganic elements can boost the sensitivity of organic components. Highly sensitive resists require less exposure time, translating to improved processing efficiencies. The team, led by Nam, has since been exploring hybrid resists with a variety of material compositions. In addition to exploiting x-ray characterization techniques at Brookhaven’s National Synchrotron Light Source II (NSLS-II)—also a DOE Office of Science User Facility—to understand these nanocomposites, they are actively engaging with leading companies in the semiconductor industry such as Intel and Samsung and collaborating with the Center for X-ray Optics at DOE’s Lawrence Berkeley National Laboratory.
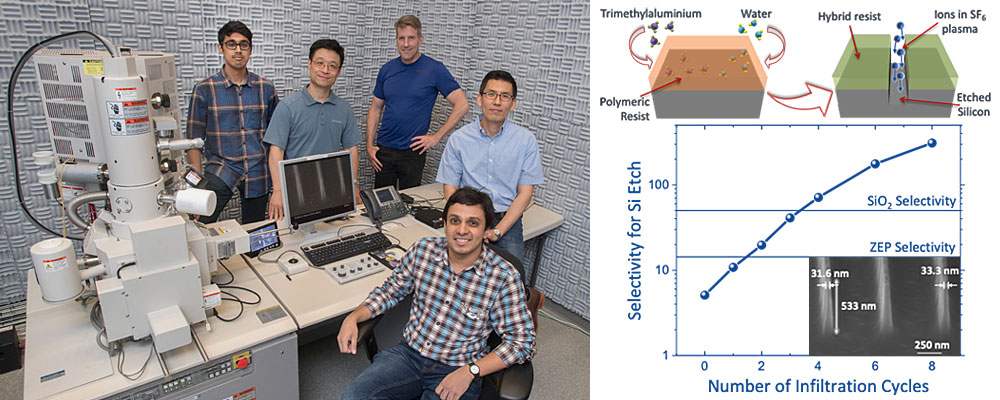
(Left to right) Ashwanth Subramanian, Ming Lu, Kim Kisslinger, Chang-Yong Nam, and Nikhil Tiwale in the CFN Electron Microscopy Facility. The team created a hybrid organic-inorganic resist through infiltration synthesis, patterned the resist via electron-beam lithography, and etched the pattern into silicon by bombarding the silicon surface with ions of sulfur hexafluoride, or SF6 (top right). The high-magnification scanning electron microscope image (inset in graph) shows high-resolution, high-aspect-ratio silicon nanostructures patterned at a pitch resolution (width of lines and the spaces between them) of 500 nanometers. As shown in the graph, after two processing cycles, the etch selectivity of the hybrid resist surpasses that of a costly resist called ZEP; after four cycles, the hybrid resist has a 40-percent-higher etch selectivity than that of silicon dioxide (SiO2).
Tiwale is now extending infiltration synthesis to new classes of semiconducting materials with unique properties that could increase computer speed and memory capabilities. He is also making ultrathin layers—only a few nanometers thick—of metal oxides through atomic layer deposition. These ultrathin layers are generated during a sequential process in which precursors react to form the desired products. Currently, Tiwale is studying and exploring how the metal oxides can be coupled with 2-D materials to make functional devices.
Lithography continues to be an important part of Tiwale’s research. Before Tiwale arrived at the CFN, the group had been combining EBL and block copolymer lithography to obtain specific morphologies in selected areas on a substrate. Block copolymers are a special class of materials where two or more chemically distinct sequences (“blocks”) spontaneously form ordered nanostructures of a particular morphology. By mixing together different block copolymers and directing them with EBL, Stein and CFN Electronic Nanomaterials Group Leader Kevin Yager were able to get lines and dots to coexist in pre-designated locations. Stein presented this work at a “Three-beams” conference that Tiwale attended during his PhD, spurring his interest.
“Morphology is important because it can dictate material properties,” explained Tiwale. “Specific arrangements of different morphologies are widely applied in photonic waveguides, semiconducting lasers, and flat lenses that are enabling the integration of complex optics into smartphone cameras.”
Collaborating with fellow postdocs in the Electronic Nanomaterials Group, Tiwale has been expanding this lithography approach to obtain a wider range of morphology types—such as holes and sheets—on the same layer. Last year, he helped with a project led by Yager in which they performed lithography multiple times to specify regions of desired morphologies on a single substrate. The team is now aiming to replace these multiple patterning steps with one step, which could make the process practically viable for fabricating device-related structures.
“If we can simultaneously direct block copolymers to assemble in a certain way at specific locations, then we will be able to make very high-density nanopatterns with function-specific morphologies—for example, lines for circuit elements, dots and holes to interconnect device circuit layers, and sheets to construct new transistor architectures. High density means we can fit more devices in the same physical space, thus making computers faster.”
Beyond his own research projects, Tiwale is collaborating with colleagues from his PhD days—now faculty at prominent research institutes in India—who have become CFN users. Currently, he is contributing to nanopatterning for next-generation solar cells based on hybrid perovskites—alternative materials to silicon that could provide higher efficiency—and making perovskite transistors to understand how electrical charges are transferred in these materials. He is also collaborating with University of Wisconsin–Madison users who are growing arrays of graphene nanoribbons that weave into each other to make large-area meshes. Graphene in its 2-D form is a metal-like conducting material, but graphene nanoribbons less than 10 nanometers wide show semiconducting behavior, making them promising channel materials for transistors.
“The CFN has provided an environment for me to not only progress my own research with the help of experts and unique capabilities, but also to expand my collaborations and drive different research ideas toward applications,” said Tiwale.
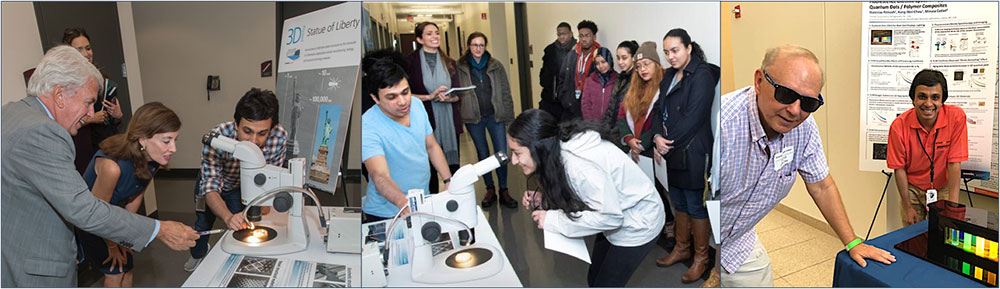
Tiwale enjoys sharing research with others through various outreach activities at the CFN, including tours and open-house events.
When Tiwale completes his postdoc next year, he would like to continue this application-oriented research and translate discoveries into practical device platforms.
“So far, semiconductor technology has been heavily dependent on advancing a particular type of device architecture to make smaller and faster devices,” said Tiwale. “But opportunities for creating different types of nanostructuring using lithography will open up as new avenues for next-generation computing—such as quantum electronics and photonic computing—are pursued and as consumer electronics head toward more interactive display platforms. I am excited to pursue these opportunities, applying my passions of science and art.”
Brookhaven National Laboratory is supported by the U.S. Department of Energy’s Office of Science. The Office of Science is the single largest supporter of basic research in the physical sciences in the United States and is working to address some of the most pressing challenges of our time. For more information, visit https://energy.gov/science.
Follow @BrookhavenLab on Twitter or find us on Facebook.
2020-17321 | INT/EXT | Newsroom