Transferring Information at the Speed of "Magnons"
Scientists at Brookhaven National Laboratory uncovered a previously unknown spectrum of magnon excitations in an antiferromagnetic thin film
August 3, 2023
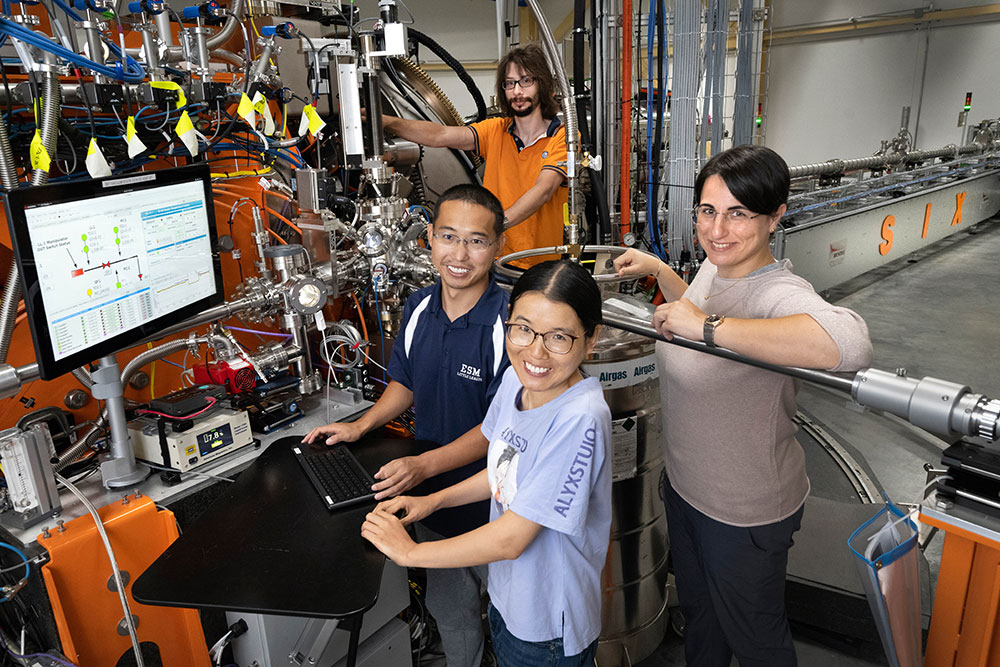
The research team at the SIX beamline. Pictured from left to right are (bottom row) Jiemin Li, Yanhong Gu, Valentina Bisogni, and (top row) Jonathan Pelliciari.
The Zoom screen flickered, just for a second, as Valentina Bisogni, Jonathan Pelliciari, and Jiemin Li, three scientists at the National Synchrotron Light Source II (NSLS-II), shared their excitement about the multi-magnon excitations they discovered. Their findings were recently published in Physical Review X.
“The magnon excitations we discovered are interesting for applications in information transfer because they don’t depend on—,” Pelliciari said, just before the screen froze and the rest of his explanation was lost in cyberspace.
“Johnny, please say that again. You froze,” Bisogni said.
“This is why we need to develop devices that use magnon excitation—so that we can transfer information without these interruptions,” said Li, with a smile.
To transfer information on a computer chip, electrons must move like runners on a familiar but slightly bumpy track. The longer the distance is, the longer the transfer takes, and the greater the chance of losing information and dissipating energy. Devices that use magnon waves to transfer information wouldn’t move electrons at all. Instead, they would use a property of the electron, called spin, to transport information. This is why this technology is called “spintronics.”
An electron’s spin can have different directions. Other electrons can “see” the spin direction of their neighbors as if they were holding a flag. When information needs to be transferred, the electron either raises or lowers its flag. The next electron mimics the movement, then the next, and so on. In this way, the information—or the change in flag position—is passed from electron to electron without any of the electrons having to move.
The research team, led by Bisogni, is working to understand the fundamental behavior of the materials that are needed to build spintronics. In this study, they focused on a well-known material called hematite, a form of iron oxide, or rust.
“In the past, hematite has mostly been studied in larger samples (called bulk) or in crystal form,” said Bisogni. “So, it is not a new material. But its potential application in spintronics is new. We are now studying this material in the form in which it would be used in potential devices. If you want to make a small device, you have to use the material as a thin film. And we know from other materials that some properties, such as magnon transfer, change depending on the shape of the material.”
Hematite has been studied in the past because it belongs to an interesting class of materials called antiferromagnets.
“Your everyday refrigerator magnet is a ferromagnet. It has a magnetic field that we can observe,” said Li. “In ferromagnets, all the spins point in the same direction and create a magnetic field. In antiferromagnets, the spins point in different directions, so there is no field that we can observe. But we can see them at the microscopic level.”
But it’s not easy to “see” spins in antiferromagnetic materials, especially if you want to see how the spins create different magnon waves. The team needed a special, one-of-a-kind research tool to measure the behavior of magnon waves in their material. That tool was the Soft Inelastic X-ray Scattering (SIX) beamline at NSLS-II. NSLS-II is a U.S. Department of Energy (DOE) Office of Science User Facility at DOE’s Brookhaven National Laboratory. The facility has 29 operational beamlines that enable researchers to study all types of materials. A beamline is a combination of an x-ray photon delivery system and an experimental endstation. A half-mile circular accelerator powers the generation of the ultrabright x-rays that are the central tool for understanding the inner workings of materials such as hematite.
Of all the impressive research tools at NSLS-II, the SIX beamline is unique in that the experimental setup is housed in a separate building. Inside this building, a 49-foot-long arm moves the detector around the sample to collect the scattered x-rays after they hit it. In this case, the sample was the thin film of hematite, but SIX can be used to study all kinds of other solid materials.
“Using the SIX beamline, we were able to measure the spectrum of the magnon waves. The first mode we found was the same as it would be in bulk material. That may not sound interesting, but it is, because it didn’t have to be the same. In our previous work on a ferromagnetic material, we found that the first modes were different for thin films and bulk. In addition, we were also able to measure higher modes that we had never seen before,” said Bisogni.
Pelliciari added, “This is also true for bulk measurements of the material. You would not be able to observe these modes because of a conceptual limitation of other techniques that are used to study spins in bulk. Most other techniques can only see up to the second excitation, but they can’t go any further.”
The modes, or excitations, are analogous to the spin positions of the electrons in the materials, or the positions of the flags mentioned earlier, and they have characteristic energies specific to the system. Knowing what energy a spin mode corresponds to leads researchers and engineers to design better devices.
Detecting these higher-order modes is a real challenge, and the team attributes the success to an impressive combination of good intensity and energy resolution from the beamline, which is improved by the length of the spectrometer arm on which the detector sits.
“The other thing that made this measurement possible is our triple-rotating flange. It allows us to rotate the spectrometer arm without breaking the vacuum connection to the sample chamber, which means we can get a more complete picture of the studied system from different geometries,” Li said.
When the x-rays hit the sample, they scatter like water on an uneven surface and fly off in different directions. Based on these directions, the researchers can learn something about the material’s internal structure. In this case, it gives them information about the spin propagation directions of the electrons. So, the triple-rotating flange enables the researchers to find out the directions the x-ray scatter by moving around the spectrometer with respect to the sample.
“One important thing that we also learned from our measurement is that the process behind the multi-magnon excitations is complex. And because it is complex, our collaboration with Atshushi Hariki and Jan Kuneš has been essential to unravel—from a theoretical point of view—the mechanism behind all the interactions at play,” Bisogni said.
The team looks forward to studying more materials using the SIX beamline at NSLS-II and other tools that will help them continue to solve this puzzle.
The study’s research team was led by Valentina Bisogni and included Jiemin Li, Yanhong Gu, Yoshihiro Takahashi, Keisuke Higashi, Taehun Kim, Yang Cheng, Fengyuan Yang, Jan Kuneš, Jonathan Pelliciari, and Atsushi Hariki.
This study was supported by U.S. Department of Energy (DOE), Office of Science, Basic Energy Sciences, Early Career Award Program, as well as Ohio State University and JSPS KAKENHI.
Brookhaven National Laboratory is supported by the Office of Science of the U.S. Department of Energy. The Office of Science is the single largest supporter of basic research in the physical sciences in the United States and is working to address some of the most pressing challenges of our time. For more information, visit science.energy.gov.
Follow @BrookhavenLab on social media. Find us on Instagram, LinkedIn, Twitter, and Facebook.
2023-21075 | INT/EXT | Newsroom