Turning to Trees for Improving Battery Performance
Scientists designed a new solid ion conductor that combines copper with polymer chains of cellulose nanofibrils
December 31, 2021
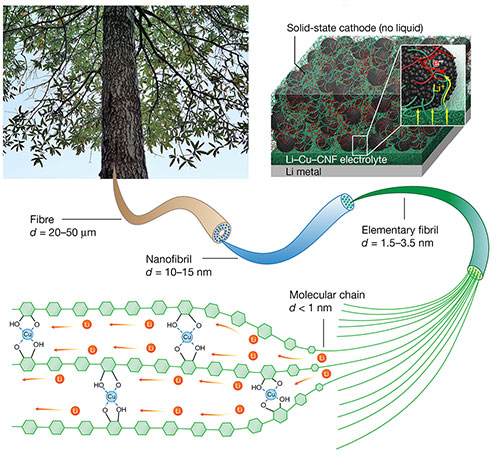
The schematic depicts how synthesized cellulose combined with copper can be used to funnel lithium ions (bottom) so that it can be used as an electrolyte in a solid-state battery (top, right). Image credit: Nature 598 (7882), 590-596 (2021)
The Science
Scientists designed a new solid ion conductor that combines copper with polymer chains of cellulose nanofibrils derived from wood for solid-state batteries.
The Impact
To replace the liquid conductors commonly used in today’s lithium-ion batteries with solid materials, researchers need to find new materials that fulfill the demand for high conductivity. This study offers a new potential candidate.
Summary
From cell phones to electric cars, our modern society relies on energy storage through batteries for many different applications. However, the demand for more energy under safer and more reliable conditions forces researchers to search for a replacement of the liquids commonly used in today’s lithium-ion batteries. Most research teams are looking at solid materials that are less flammable and more cost-effective for this endeavor.
In this study, the research team has developed a new material for use in solid-state batteries that is derived from trees. More precisely, the research team demonstrated the effectiveness of a new solid ion conductor that combines copper with cellulose nanofibrils, which are polymer tubes derived from wood. The new material is paper-thin and has a 10 to 100 times better conductivity than other polymer ion conductors. The researchers showed that it could be used as either a solid battery electrolyte or as an ion-conducting binder for the cathode of an all-solid-state battery. By incorporating copper with one-dimensional cellulose nanofibrils, the team transformed the normally ion-insulating cellulose into a lithium-ion transporter within the polymer chains.
The researchers used a number of research techniques to investigate the detailed behavior of the new material on its own but also as part of a battery. To study the behavior of the copper inside the cellulose, the team turned to the Inner Shell Spectroscopy (ISS) beamline at the National Synchrotron Light Source II (NSLS-II). By using the ISS beamline and working with NSLS-II staff, the team verified the behavior and position of the copper atoms within the new material. The ISS beamline is part of a suite of advanced x-ray spectroscopy tools available to researchers for various studies in materials science at NSLS-II, which is a U.S. Department of Energy (DOE) Office of Science User Facility located at DOE’s Brookhaven Lab.
In addition to their experimental studies, the team performed computer simulations on the microscopic structure of the new material to understand why it is able to conduct ions so well. The model showed that the copper widens the space between cellulose polymer chains, which are normally tightly packed together. This expanded spacing creates a channel through which lithium ions can flow relatively unimpeded.
The researchers hope that the new material is a path forward for mass producing solid-state batteries.
Download the research summary slide (PDF)
Related Links
News Story: New Material Could Pave Way for Better, Safer Batteries
Contact
Liangbing Hu
University of Maryland
binghu@umd.edu
Yue Qi
Brown University
yueqi@brown.edu
Publications
Chunpeng Yang, Qisheng Wu, Weiqi Xie, Xin Zhang, Alexandra Brozena, Jin Zheng, Mounesha N. Garaga, Byung Hee Ko, Yimin Mao, Shuaiming He, Yue Gao, Pengbo Wang, Madhusudan Tyagi, Feng Jiao, Robert Briber, Paul Albertus, Chunsheng Wang, Steven Greenbaum, Yan-Yan Hu, Akira Isogai, Martin Winter, Kang Xu, Yue Qi & Liangbing Hu. Copper-coordinated cellulose ion conductors for solid-state batteries. Nature 598 (7882), 590-596 (2021). 10.1038/s41586-021-03885-6
Funding
L.H. and C.Y. acknowledge support from the University of Maryland A. James Clark School of Engineering. Q.W. and Y.Q. acknowledge support from the National Science Foundation (NSF) under grant DMR-2054438. Y.-Y.H. acknowledges support from the NSF under grant DMR-1847038. Solid-state NMR experiments were carried out at the National High Magnetic Field Laboratory, which is supported by the NSF through NSF/DMR-1644779 and the State of Florida. The NMR diffusion work at Hunter College was supported in part by the US Office of Naval Research, grant N00014-20-1-2186. This research used resources at the 8-ID Beamline of the National Synchrotron Light Source II, a US Department of Energy Office of Science User Facility operated by Brookhaven National Laboratory under contract no. DE-SC0012704. Access to the High Flux Backscattering Spectrometer was provided by the Center for High Resolution Neutron Scattering, a partnership between the National Institute of Standards and Technology (NIST) and the NSF under agreement DMR-1508249. Certain commercial equipment, instruments and materials are identified in this paper in order to specify the experimental procedure adequately. Such identification is not intended to imply recommendation or endorsement by the NIST. K.X. acknowledges support from the Joint Centre for Energy Security Research (JCESR), an energy hub funded by the US Department of Energy, Office of Science, Basic Energy Science under IAA SN2020957. We thank F. Lin, D. Hou and Z. Yang from Virginia Tech for providing the NMC811 cathode material.
2021-20630 | INT/EXT | Newsroom