Pathway to a New Brønsted Acid Catalyst
Scientists demonstrate how a new Brønsted acid catalyst could improve petrochemical, fine chemical, or pharmaceutical upgrade reactions
June 8, 2022
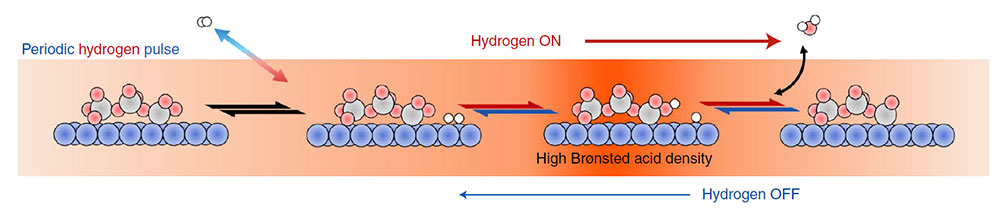
The schematic shows how hydrogen pulses can sustain high Brønsted acid density. Image credit: Nature Catalysis 5, 144–153
The Science
Scientists demonstrated how the acidity of a catalyst can be modulated by changing the pretreatment conditions and by periodic hydrogen pulsing, causing a dramatic increase in the rate of the acid-catalyzed reaction.
The Impact
Inverse catalysts such as Brønsted acid—consisting of oxide clusters on metal—are efficient for petrochemical, fine chemical, pharmaceutical, and biomass upgrade reactions. This study offers new insights into these catalysts’ active sites and how to tune them.
Summary
From petrochemical to pharmaceutical upgrade reactions, metal-to-metal catalysts play an important role due to their multiple active sites. Brønsted acid sites offer a new pathway to developing a new catalyst for such reactions. However, the nature and dynamics of Brønsted acid sites under working conditions remain poorly understood due to the functionalities of the constituent materials.
In this study, a team of researchers demonstrated Brønsted acid catalysis as well as a number of essential parts of the reaction such as water dissociation, hydrogen spillover, and oxide reduction. The team synthesized the catalyst by atomic layer deposition (ALD) and used density functional theory (DFT) calculations, microkinetic modeling (MKM), and experimental kinetics with probe molecules to elucidate the structure, catalyst phase behavior, and the dynamics of the acid sites under various working conditions. As part of this extensive study, the team collaborated with Brookhaven Lab researchers at the Center for Functional Nanomaterials (CFN) and the National Synchrotron Light Source II (NSLS-II). At NSLS-II, the team used the Quick X-ray Absorption and Scattering (QAS) beamline for time-dependent x-ray studies on their catalyst, while at the CFN, the group used the Ambient Pressure X-ray Photoelectron Spectroscopy system (part of the CFN Proximal Probes Facility) to study the reduction of oxygen during the reaction.
The fundamental insights from this study could enable the development of more efficient inverse catalysts.
The CFN and NSLS-II are U.S. Department of Energy (DOE) Office of Science user facilities located at DOE’s Brookhaven National Laboratory.
Download the research summary slide (PDF)
Contact
Dionisios G. Vlachos
University of Delaware
vlachos@udel.edu
Publications
Jiayi Fu, Shizhong Liu, Weiqing Zheng, Renjing Huang, Cong Wang, Ajibola Lawal, Konstantinos Alexopoulos, Sibao Liu, Yunzhu Wang, Kewei Yu, J. Anibal Boscoboinik, Yuefeng Liu, Xi Liu, Anatoly I. Frenkel, Omar A. Abdelrahman, Raymond J. Gorte, Stavros Caratzoulas & Dionisios G. Vlachos Modulating the dynamics of Brønsted acid sites on PtWOx inverse- catalyst. Nature Catalysis 5, 144–153 (2022). [DOI: 10.1038/s41929-022-00745-y]
Funding
This work was financially supported primarily by the Catalysis Center for Energy Innovation, an Energy Frontier Research Center funded by the US Department of Energy, Office of Science, Office of Basic Energy Sciences under award number DESC0001004. Portions of this work were performed at the DuPont–Northwestern–Dow Collaborative Access Team (DND-CAT) located at Sector 5 of the Advanced Photon Source. DND-CAT is supported by Northwestern University, E.I. DuPont de Nemours and Co. and The Dow Chemical Company. This research used resources of the Advanced Photon Source, a US Department of Energy, Office of Science user facility operated for the Department of Energy, Office of Science by Argonne National Laboratory under contract no. DE-AC02-06CH11357. The research was carried out in part at the 7-BM (QAS) beamline of the National Synchrotron Light Source II at Brookhaven National Laboratory, which is supported by the Synchrotron Catalysis Consortium, US Department of Energy under grant no. DE-SC0012335 and at the Center for Functional Nanomaterials, Brookhaven National Laboratory, which is supported by the US Department of Energy, Office of Basic Energy Sciences under contract no. DE-SC0012704. Some of the XPS measurements were carried out using the Thermo Scientific K-Alpha+ XPS System at the University of Delaware surface analysis facility supported by NSF (no. 1428149). Y.L. acknowledges financial support from the Chinese Academy of Science Youth Innovation Promotion Association (no. 2018220) and LiaoNing Revitalization Talents Program (no. XLYC1907053), and X.L. acknowledges financial support from the National Natural Science Foundation of China (nos. 21872163, 22072090, 21991153 and 21991150) for the annular dark-field (ADF)-STEM analysis. The authors acknowledge H. Matsumoto for assistance with STEM data acquisition from a Hitachi HF5000 microscope. We acknowledge W. Wu for assisting with the XPS measurements, Q. Ma, S. Ehrlich, N. S. Marinkovic and L. Ma for helping with the XAS measurements and S. Prodinger for valuable discussions.
2022-20687 | INT/EXT | Newsroom