Sulfur Iodide Paves the Way for Cheaper, 'Healable' Vehicle Batteries
August 30, 2024
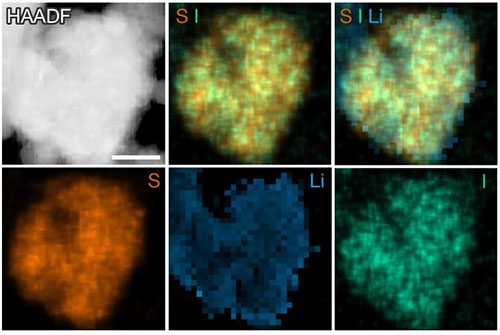
Cryo-TEM HAADF (high-angle annular dark-field), EDX, and EELS mapping images of S9.3I. The S and I maps are acquired using EDX, the Li map is acquired using EELS. The scale bar is 200 nm. Credit: Nature 627, 301-305 (2024).
The Science
By adding iodine (I) to a solid-state lithium sulfur (Li-S) battery (SSLSB), scientists yielded vastly improved conductivity and a low melting point that promotes self-repair of interfaces.
The Impact
The results may help realize SSLSBs as a viable option for more cost-effective and robust electric vehicle batteries.
Summary
Solid-state lithium-sulfur (Li–S) batteries (SSLSBs) have always sounded promising in theory but have proven difficult to create practically. SSLSBs can be made with inexpensive and widely available materials that have a theoretically high energy density, making them an attractive battery for electric vehicles, but there are still challenges to address before this new technology can be realized. For example, previous work found that sulfur’s insulating nature creates issues with conductivity and charge transfer. To address this, a research team led by the University of California, San Diego, La Jolla added iodine to the crystalline sulfur structure. They found that this combination can instill SSLSBs with semiconductor-level electrical conductivity, magnitudes above sulfur alone. Another benefit of the iodine addition is that the combined material now has a lower melting point, which promotes self-repair to damage that occurs at the battery interfaces over multiple charge-discharge cycles.
Several beamlines at the National Synchrotron Light Source II (NSLS-II), a U.S. Department of Energy (DOE) Office of Science User Facility at DOE’s Brookhaven National Laboratory, were used to observe and characterize this conductive, low-melting-point sulfur iodide material. The melting point was confirmed as the material was heated using in situ X-ray diffraction at the X-ray Powder Diffraction (XPD) beamline. The Quick x-ray Absorption and Scattering (QAS) and Tender Energy X-ray Absorption Spectroscopy (TES) beamlines were able to provide X-ray absorption spectroscopy data to give researchers a better idea of the materials electrical structure and what was happening as it was being charged. Additional techniques used include cryo-transmission electron microscopy (cryo-TEM) imaging, energy-dispersive X-ray spectroscopy (EDX), and electron energy loss spectroscopy (EELS).
These studies demonstrated that batteries made with this material last for 400 cycles with only a minimal loss of capacity, making them a viable option for more cost-effective and robust electric vehicles.
Download the research summary slide (PDF)
Contact
Shyue Ping Ong
University of California, San Diego
ongsp@ucsd.edu
Ping Liu
University of California, San Diego
piliu@ucsd.edu
Publications
J. Zhou, M.L. Holekevi Chandrappa, S. Tan, W. Shen, C. Wu, H. Nguyen, C. Wang, H. Liu, S. Yu, Q.R.S. Miller, G. Hyun, J. Holoubek, J. Hong, Y. Xiao, C. Soulen, Z. Fan, E.E. Fullerton, C.J. Brooks, C. Wang, R.J. Clément, Y. Yao, E. Hu, S.P. Ong, P. Liu. “Healable and conductive sulfur iodide for solid-state Li-S batteries.” Nature 627, 301-305 (2024). DOI: 10.1038/s41586-024-07101-z
Funding
This work was supported by the Advanced Research Projects Agency–Energy, US Department of Energy (DOE), under contract no. DE-AR0000781. M.L.H.C. and S.P.O. acknowledge the support from the Materials Project, funded by the US DOE, Office of Science, Office of Basic Energy Sciences, Materials Sciences and Engineering Division, under contract no. DEAC02-05-CH11231 (Materials Project Program No. KC23MP). Computing resources were provided by the National Energy Research Scientific Computing Center (NERSC) and the Advanced Cyberinfrastructure Coordination Ecosystem: Services & Support (ACCESS) under grant no. DMR-150014. S.T. and E.H. are supported by the Assistant Secretary for Energy Efficiency and Renewable Energy (EERE), Vehicle Technology Office of the US DOE through the Advanced Battery Materials Research (BMR) Program under contract no. DE-SC0012704. This research used 28-ID−2, 8-BM and 7-BM beamlines of the National Synchrotron Light Source II, US DOE Office of Science User Facilities, operated for the DOE Office of Science by Brookhaven National Laboratory under contract no. DE-SC0012704. C. Wu, Z.F. and Y.Y. are supported by the US DOE’s Office of EERE under the Vehicle Technologies Program under contract no. DE-EE0008864. This work made use of the shared facilities of the UC Santa Barbara MRSEC (grant no. DMR-720256), a member of the Materials Research Facilities Network (http://www.mrfn.org). This research used the Electron Microscopy facility of the Center for Functional Nanomaterials (CFN), which is a US DOE Office of Science User Facility, at Brookhaven National Laboratory, under contract no. DE-SC0012704. Canhui Wang and Chao Wang were supported by the Advanced Research Projects Agency–Energy, US DOE, under contract no. DE-AR0001191. FIB and SEM characterizations were performed at the San Diego Nanotechnology Infrastructure (SDNI) of UCSD supported by the NSF (grant no. ECCS1542148). Raman facilities were supported by the NSF through UCSD MRSEC, grant no. DMR-201192. The authors acknowledge the use of facilities and instrumentation at the UC Irvine (UCI) Materials Research Institute (IMRI), which was supported in part by the NSF through the UCI MRSEC (grant no. DMR-2011967). XPS facilities were funded in part by the NSF Major Research Instrumentation. We thank the Molecular Mass Spectrometry Facility at UC San Diego for performing the MALDI-TOFMS measurement, which is supported by the NSF under grant no. CHE−1338173. Part of this work was performed in the Cordx Yufeng Li Collaboratory.
2024-22072 | INT/EXT | Newsroom