Quantum Network Facility
Availabile Equipment
The facility is equipped with the state-of-the-art quantum networking equipment necessary to build long distance entanglement distribution networks.
- Single Photon Detection
- Hong-Ou-Mandel Interference and Bell State Measurements
- Portable Quantum Memory and Phase Lock Laser System
- Telecom-Band Entangled Photon Sources
- Multiplexed Quantum Memories and Distributed Quantum Sensors
- Telecom Laser Infrastructure
- Tunable Infrared Laser and Second Harmonic Generation
- Enabling Technologies for Long Distance Quantum Communication
- Quantum-Assisted Astronomy/Imaging Projects
Single Photon Detection
The facility has two operational Superconducting Nanowire Single Photon Detector (SNSPD) systems. The first comprises four channels that are sensitive at telecom wavelengths (~1300-1500 nm) and four channels that are sensitive at near-infrared (NIR) (~800 nm). The second system, which is portable, has two telecom channels and two NIR channels.
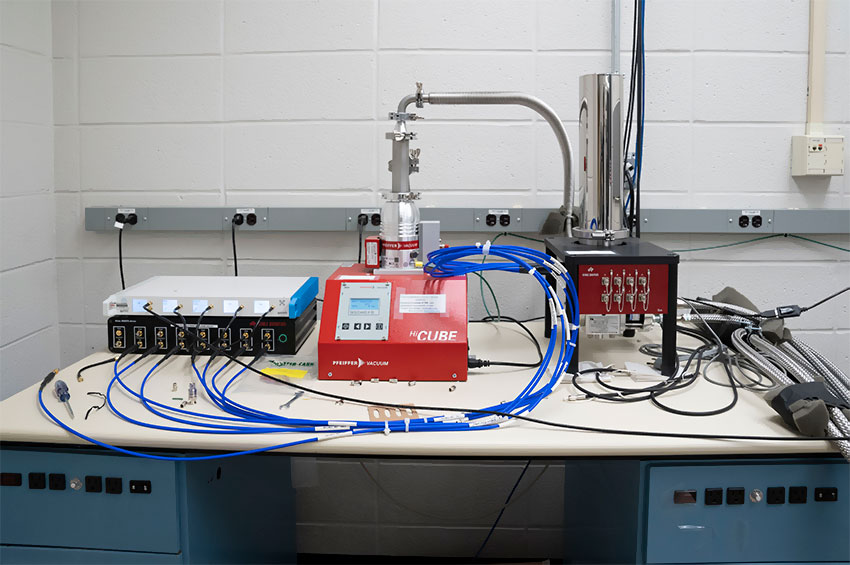
Hong-Ou-Mandel Interference and Bell State Measurements
The facility has developed an interferometric experiment designed to receive photons carrying polarization qubits and entanglement at telecom wavelengths. The setup allows researchers to perform quantum state tomography or measurement-based entanglement swapping. Direct connection to commercial fibers in the Long Island optical fiber network is also available.
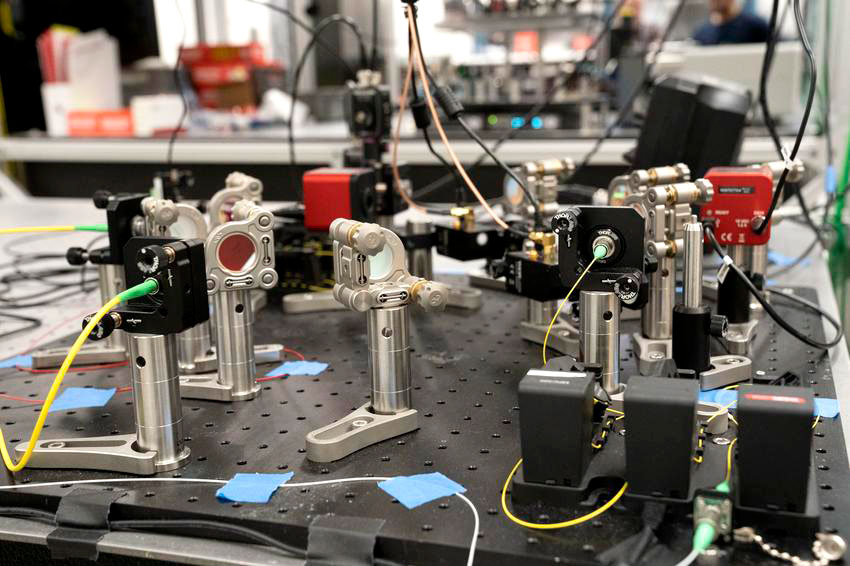
Portable Quantum Memory and Phase Lock Laser System
The facility possesses a first-of-its-kind, room temperature, rack-mounted quantum memory built by Qunnect Inc. It is used to store polarization qubits at a wavelength of 795 nm and is compatible with fiber operation.
The same rack houses a 795 nm, high-power, tapered, amplified laser that is tunable to atomic transitions of rubidium atoms. This laser can be used for the creation of Raman pairs that drive atomic coherence effects, such as Electromagnetic-Induced Transparency (EIT), to drive the memory. The facility also has laser electronics, including phase detectors and Proportional-Integral-Derivative controllers, that permit phase locking the laser to another laser system.
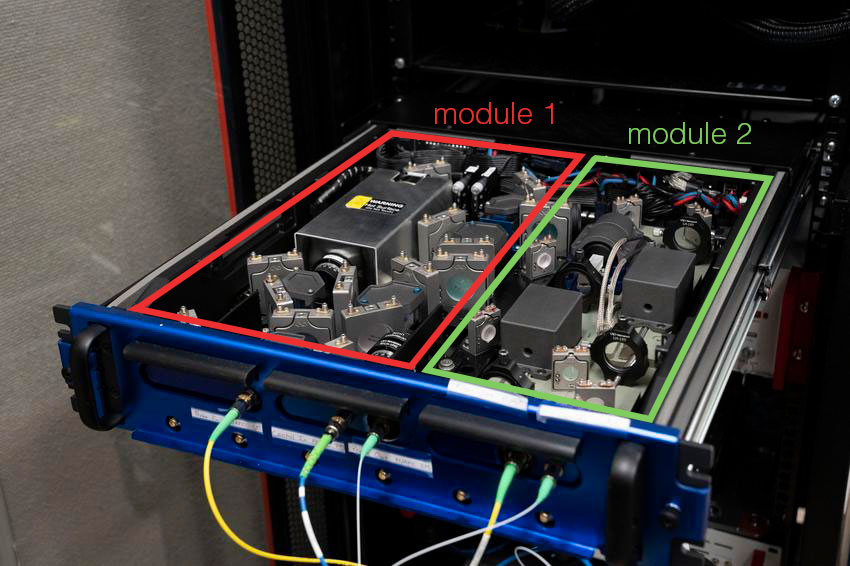
Rack-mounted, commercially manufactured, portable rubidium (Rb) vapor quantum memory system. Module 1: Rb vapor quantum memory, with control and probe field optical inputs and readback output. Module 2: Ultra narrow-band filter to separate control field from the single photon-level probe field (output field).
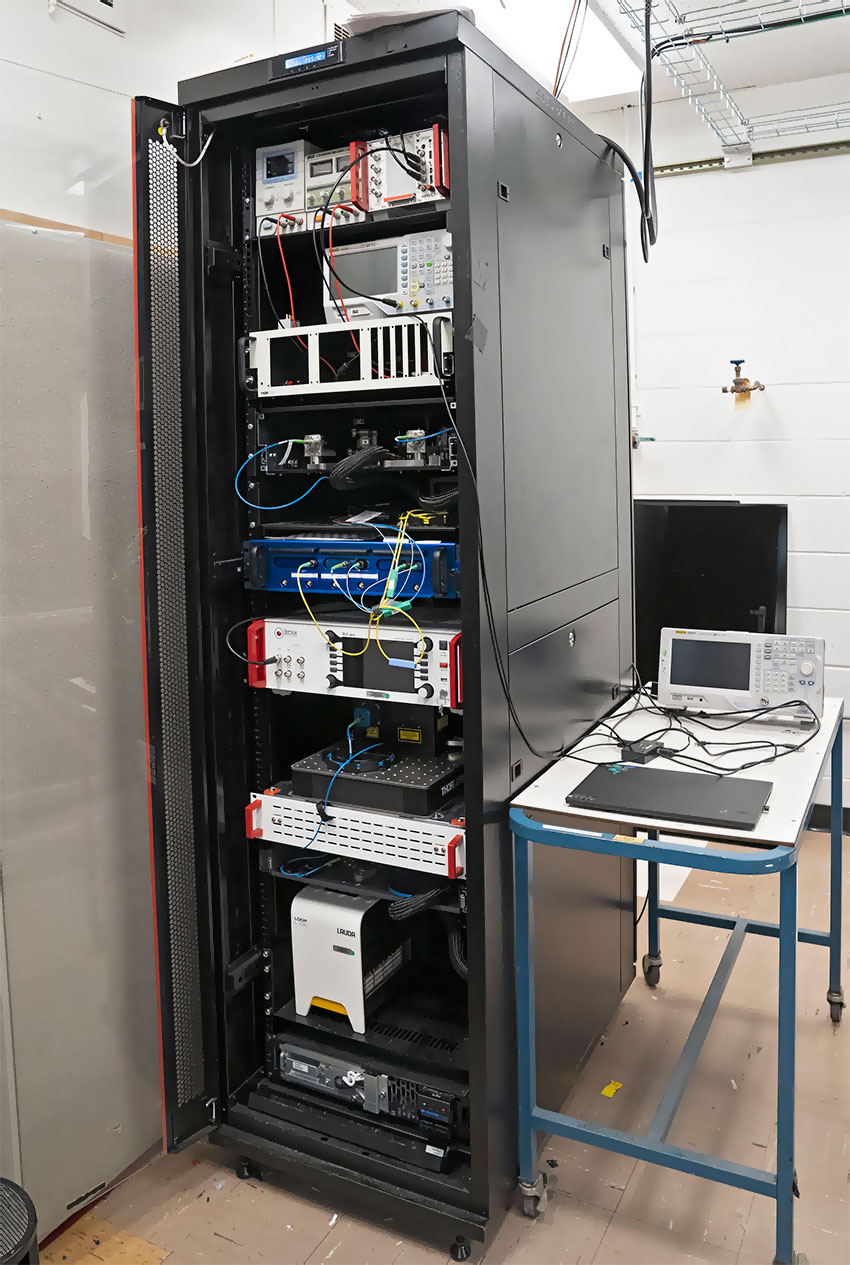
Telecom-Band Entangled Photon Sources
Currently, the facility is developing a polarization entanglement source operational at telecom wavelengths. In this source, an input pump laser tuned to a wavelength of 622 nm is used to generate photon pairs centered around the 1324 nm 5P1/2 -> 6S1/2 transition of rubidium atoms. Polarization entanglement is achieved with two (Periodically Poled Lithium Niobate) PPLN non-linear Type-I crystals that are concatenated to produce a Bell state of the form |HH> + |VV>. Currently, we are developing an upgrade to this system using Optical Parametric Amplification (OPA) to improve the rates of generated twin photon pairs at the desired wavelength.
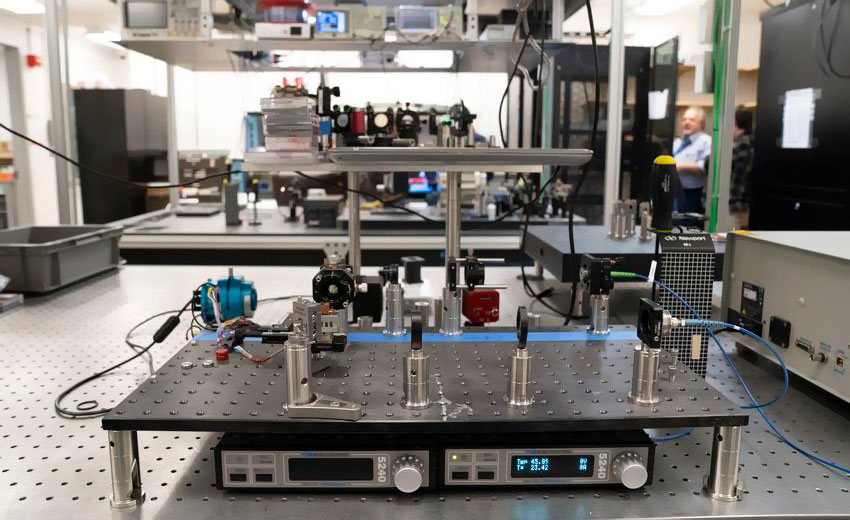
Multiplexed Quantum Memories and Distributed Quantum Sensors
The facility is developing room temperature quantum memories capable of multiplexing multiple spatial modes. The spatial multiplexing is achieved using systems of electronically driven acousto-optical deflectors (AODs) to produce light grids of laser Raman pairs that are used to create multiplexed, electromagnetically-induced transparency systems in independent regions of an atomic ensemble. This grid of local quantum memories is envisioned to be a testbed to investigate the principles of distributed quantum sensing.
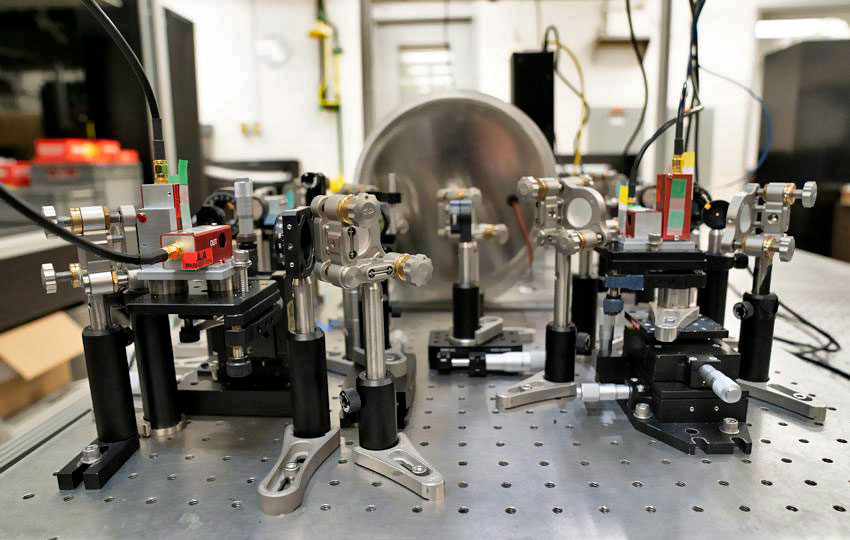
Telecom Laser Infrastructure
The facility possesses two tunable tapered-amplified telecom lasers, operational at the original band of telecom transmission. Currently, the lasers are tuned to a wavelength of 1324 nm. One of the telecom systems is integrated with a second harmonic generation system, able to produce visible radiation at a wavelength of 662 nm. These systems are used in the production of entangled photon pairs and the stabilization of cavities to enhance the rate of photon pair production. There are also specialized two-photon absorption spectroscopy setups that allow for stabilization of the tunable lasers to atomic transitions in the telecom band.
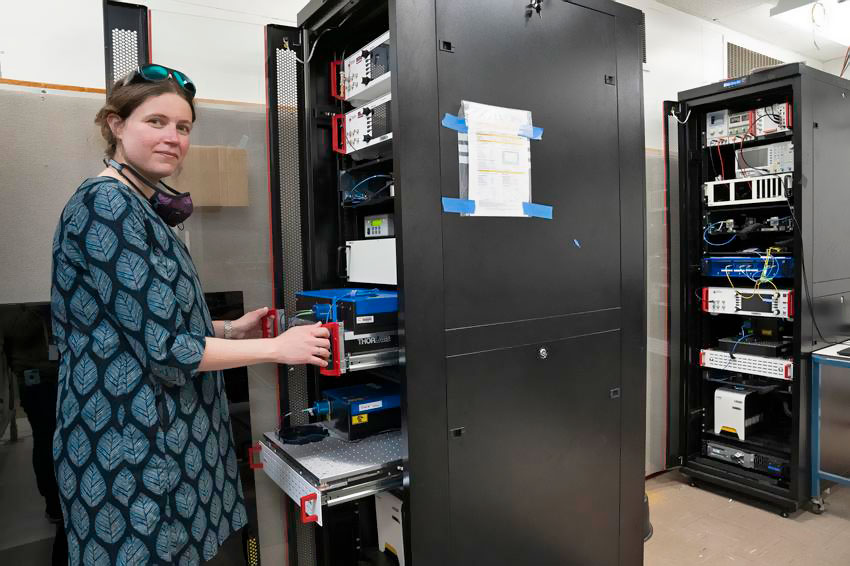
Tunable Infrared Laser and Second Harmonic Generation
The facility has the first examples of rack mounted laser technology that are the core of its portable quantum memory systems. In these rack systems, there are tunable lasers that cover the D1 and D2 (795 nm and 780 nm) lines of rubidium, and portable spectroscopy setups for laser stabilization. There is also a second harmonic frequency generator that allows conversion to a wavelength of 397 nm that is used as the pump to produce photon pairs at wavelengths compatible with quantum memory operation.
The facility also has two rack-mounted 780 nm lasers for atom cooling and, in the near future, will obtain a dilution refrigerator to perform transduction experiments between 795 nm rubidium-compatible qubits and microwave trasmon qubits.
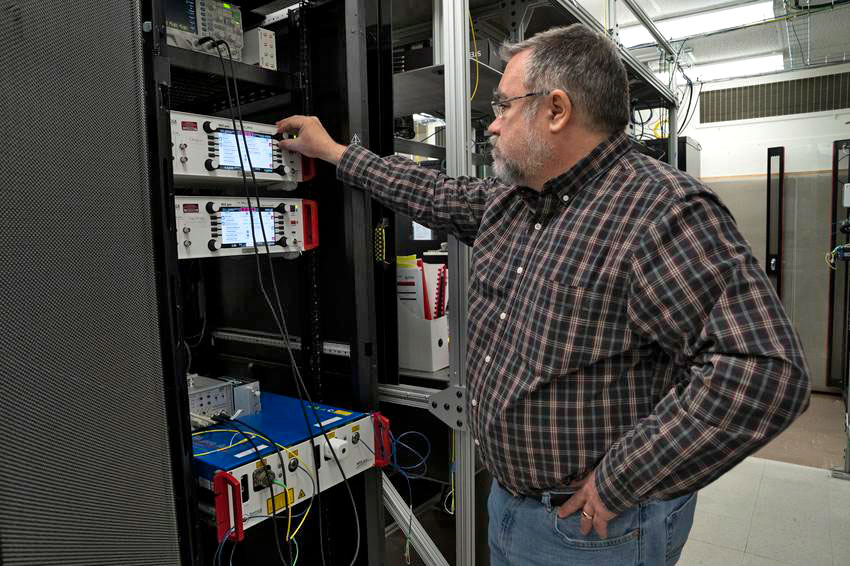
Enabling Technologies for Long Distance Quantum Communication
The Quantum Network Facility already possesses a fiber infrastructure meant for the initialization, management, and online control of the quantum hardware. It has classical communication systems, including servers for data analysis and storage, optically connected switches forming the backbone of the control networks, wave-multiplexing systems to manage the traffic of classical and quantum information in the same fibers, and White Rabbit time synchronization systems for optical clock distribution among the network nodes.
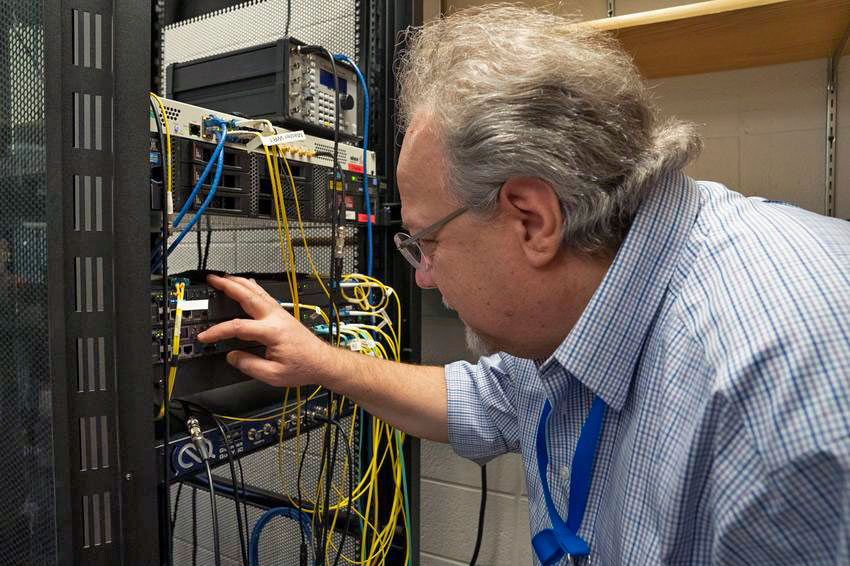
The following (rack-mounted) equipment is pictured: Quantum Composer for programming the generation of pulse trains; White Rabbit for long-distance synchronization; Dense Wavelength Division Multiplexer (DWDM) and a Filter Wavelength Division Multiplexer (FWDM) to mux/demux the necessary classical signals (timing, control, and data) into/out of one of the fiber pairs connecting Brookhaven Lab and Stony Brook University; and Q-Sync synchronized trigger generator.
Quantum-Assisted Astronomy/Imaging
A promising quantum networking technology that the Brookhaven National Laboratory Quantum Information Science (QIS) facility is exploring is improved, high-resolution astronomical imaging through optical interferometry. Many of the goals in interferometry that are hard to achieve using conventional methods, such as using very long (multi-kilometer) baselines for higher resolutions and integrating large arrays with many (100s – 1000s) apertures, could be achieved with quantum capabilities and technologies, including single-photon sources, long-distance entanglement distribution, and fast single-photon detector arrays. We have an active observational program with the goal of making precision measurements on close stellar binaries, with applications in cosmology and related fields.
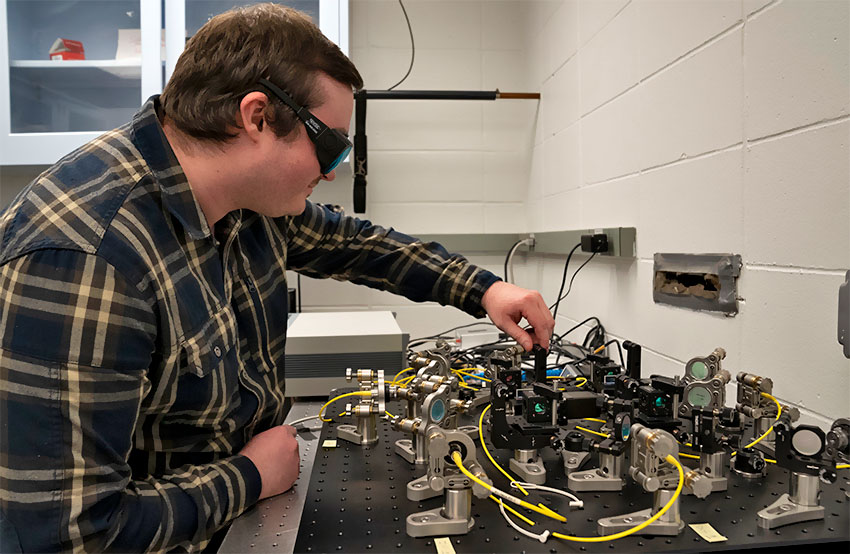
A two-input, four-beamsplitter, fiber-coupled interferometer uses narrow-band thermal light from argon lamps. This is a test bed to demonstrate two-photon amplitude interferometry, a novel technique which can then be transferred to astronomical telescopes. When successful, this approach will provide a new method for high-accuracy measurements of star positions, e.g., astrometry, as well as high-resolution imaging using arbitrarily long ground baselines.
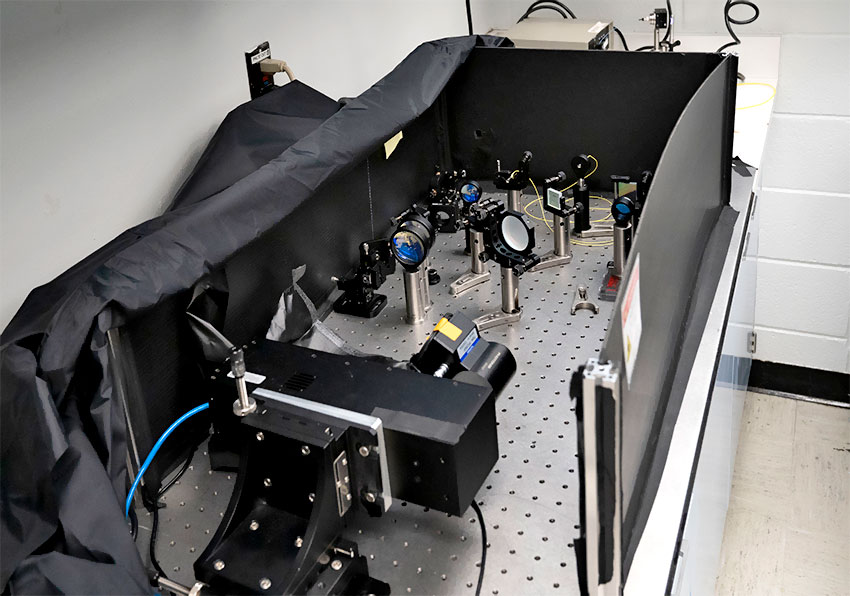
Prototype fast, fine-grained, fiber-coupled spectrograph. To achieve astronomical interferometry over multiple spectrographic bins we use a diffraction grating to spread the starlight beams across an array of pixel detector elements in a fast sensor camera. The current imager, a TimePix3 camera, provides single-photon arrival time measurement with ~1 nanosecond precision; a near-future upgrade to a SPAD array will improve that to ~150 picoseconds.