Driving Water Splitting to Create Chemical Fuels
Scientists expanded their understanding of the surface energetics and improved the conductivity of a promising electrode material for converting sunlight and water into hydrogen
December 7, 2020
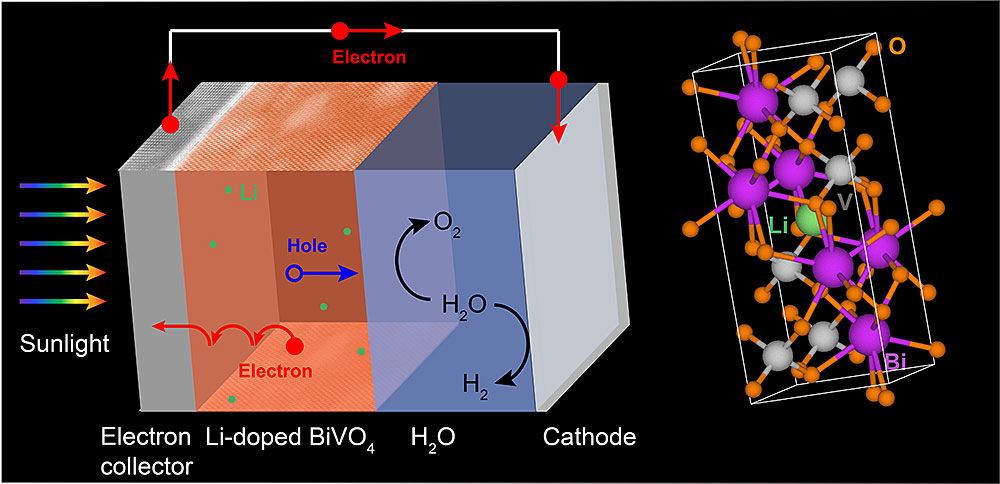
(Left) Under sunlight, bismuth vanadate (BiVO4)—a promising electrode material for water splitting—generates electron-hole pairs (charge carriers) to split water into hydrogen and oxygen gas. By "doping," or combining, bismuth vanadate with lithium (Li), scientists increased the electrical conductivity and water oxidation activity of the material. Water oxidation, which generates oxygen gas, is one of two reactions for water splitting; the second reaction generates hydrogen gas, a fuel. (Right) An atomic model of Li-doped BiVO4 shows an Li atom (green) occupying a void within the lattice.
The sun is an abundant source of renewable energy, which can be captured and converted into usable electricity. However, because the sun doesn’t always shine, the supply of energy is not continuous. We need a way to store the sun’s energy so that it can be released on demand during “off” times, such as at nighttime and in cloudy conditions.
One option is to use solar energy to power chemical reactions that generate fuels. For example, solar energy can be converted to hydrogen—an energy-dense and clean-burning fuel—through water splitting. To drive this reaction, two electrodes made of light-absorbing semiconducting materials are connected and submerged in water. Sunlight striking the electrodes creates an electric current that splits water into its two components: hydrogen and oxygen.
“We need low-cost, widely available, and environmentally friendly semiconductors that can absorb light over a range of wavelengths and efficiently carry out the oxidation of water into oxygen gas, the most challenging part of the reaction,” explained Mingzhao Liu, a staff scientist in the Interface Science and Catalysis Group of the Center for Functional Nanomaterials (CFN) at the U.S. Department of Energy’s (DOE) Brookhaven National Laboratory. “When exposed to oxygen, semiconductors can easily become corroded.”
For example, silicon, the semiconductor typically used in solar cells, quickly corrodes when exposed to oxygen. Titanium dioxide has shown high stability and electrical conductivity, but it only absorbs ultraviolet (UV) light, which accounts for only about six percent of all solar radiation received on Earth’s surface. Another promising candidate is bismuth vanadate. Made of bismuth, vanadium, and oxygen (BVO), this yellow-colored, nontoxic material has high stability and can absorb both UV and visible light. However, it’s a poor conductor of electricity, limiting its solar-to-hydrogen conversion efficiency.
Over the past few years, Liu has been leading an effort to grow high-quality BVO and enhance its performance for solar water splitting. As Liu explained, BVO is a complicated material because it’s made of two different metals and oxygen. If the ratios of atoms aren’t exactly one to one, defects can be unintentionally introduced. These defects make it difficult to study the true properties of the material and discover its inherent limitations.
To create thin films of BVO with a high purity and crystalline structure, Liu has been using pulsed laser deposition. In this technique, a focused UV laser heats a target material with the desired elemental composition inside a vacuum chamber. Because the energy of the laser pulses is very intense, atoms on the surface of the target material vaporize and condense onto a substrate to form a thin film.
“Once we have a defect-free crystalline material, then we can ask, how can we improve it?” said Liu.
In a study published earlier this year, Liu, CFN colleagues, and theorists from the University of California (UC), Santa Cruz, investigated how the electrical conductivity of BVO could be improved by adding small amounts of other materials to it (a process known as doping) via pulsed laser deposition. The theorists’ electronic structure calculations indicated that lithium would be an ideal dopant to test experimentally; lithium would easily contribute one electron to the system at room temperature and be small enough to fit inside voids within the lattice without significantly affecting its structure.
After synthesizing BVO thin films doped with an optimal amount of lithium, the team conducted a series of electron- and x-ray-based characterization studies at the CFN and Brookhaven’s National Synchrotron Light Source II (NSLS-II). These studies confirmed the purity of the films and the lack of lattice distortions following lithium doping. Then, the team measured the electronic transport properties and photoelectrochemical performance of the lithium-doped BVO. According to these experiments, lithium doping increased the conductivity of BVO by almost two orders of magnitude and its water oxidation activity by 20 percent, as compared to pure BVO.
“Theory prediction and experimental validation go hand in hand for rapidly creating new materials for energy conversion,” said Yuan Ping, an assistant professor in the Chemistry and Biochemistry Department and principal investigator of the Ping Group at UC Santa Cruz.
In another recent study, Liu and collaborators from the University of Chicago and University of Wisconsin–Madison investigated the impact of oxygen vacancies on the electronic structure and transport properties of BVO in its most energetically stable orientation. As Liu explained, sites in the lattice where oxygen is missing are inherent to oxide materials, even without doping. Using computational methods, the team created a structural model of BVO and validated this model by comparing computed and experimental electronic states. Their results suggested that oxygen vacancies in the bulk (inside) of the material contribute to conductivity, while those at the surface do not and may actually hinder conductivity.
“The oxygen vacancies at the surface act more like charge traps,” said Liu. “When charges go there, they become localized and stuck.”
Follow-on studies are needed to understand how surface oxygen vacancies and their tendency to immobilize charges are affected when BVO is immersed in water and working in conjunction with a co-catalyst to enhance charge transfer. The scientists will investigate whether transition metal oxides can effectively work as co-catalysts. They will also explore how the activity of solar water splitting depends on what type of atoms (bismuth or vanadium) terminate the surface layer.
“In both studies, the close collaborations between experimentalists and theorists was key to our success,” said Liu. “We look forward to continuing these collaborations to further expand our understanding of BVO and identify mechanisms to boost its performance.”
The lithium doping research used resources of the CFN Materials Synthesis and Characterization and Electron Microscopy Facilities; the NSLS-II Inner-Shell Spectroscopy (ISS) beamline; the Scientific Data and Computing Center, part of Brookhaven’s Computational Science Initiative; and the lux supercomputer at UC Santa Cruz and Extreme Science and Engineering Discovery Environment, both funded by the National Science Foundation (NSF). The CFN and NSLS-II are DOE Office of Science User Facilities. This research was supported by the DOE Office of Science and NSF. The surface oxygen vacancies research used resources of the CFN Materials Synthesis and Characterization, Proximal Probes, and Theory and Computation Facilities and University of Chicago’s Research Computing Center. This research was supported by the DOE Office of Science, NSF, and U.S. Army Research Office. Both studies were published in Chemistry of Materials.
Brookhaven National Laboratory is supported by the U.S. Department of Energy’s Office of Science. The Office of Science is the single largest supporter of basic research in the physical sciences in the United States and is working to address some of the most pressing challenges of our time. For more information, visit https://energy.gov/science.
Follow @BrookhavenLab on Twitter or find us on Facebook.
2020-17489 | INT/EXT | Newsroom