- Home
-
Research Groups
Division Groups
- Artificial Photosynthesis
- Catalysis: Reactivity & Structure
- Electrochemical Energy Storage
- Electron- and Photo-Induced Processes for Molecular Energy Conversion
- Neutrino and Nuclear Chemistry
- Surface Electrochemistry and Electrocatalysis
Associated Groups
- Catalysis for Alternative Fuels Production
- Nanostructured Interfaces for Catalysis
- Structure and Dynamics of Applied Nanomaterials
- People
- Operations
- News
- Events

Neutrino and Nuclear Chemistry
Current Experiments
SNO+ Neutrinoless Double Beta Decay
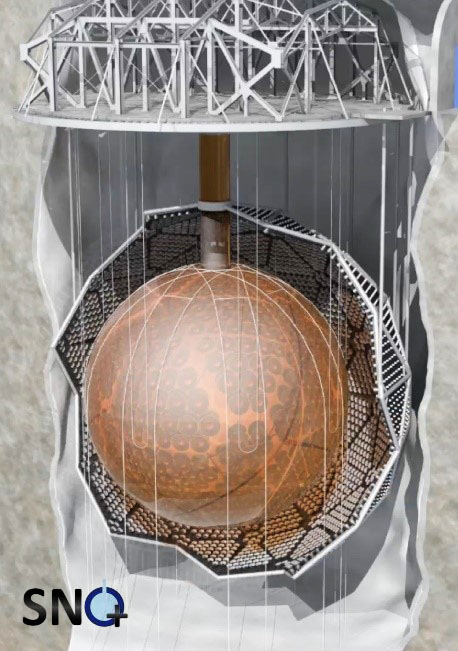
SNO+ is a large-scale liquid scintillator experiment for neutrinoless double beta decay search. The SNO+ detector is located 2km underground in Vale's Creighton mine near Sudbury, Ontario, Canada. The heart of the SNO+ detector is a 12m diameter acrylic sphere located within a cavity excavated in the rock. The vessel is filled with 860 tons of liquid scintillator and monitored by nearly 10,000 photomultiplier tubes (PMTs), which are very sensitive light detectors.
The PMT’s are supported by a geodesic stainless-steel structure. The rock cavity surrounding the detector is filled with 7000 tons of ultra-pure water, which acts as a background radiation shield. The 2092m of rock overburden shields the detector against cosmic muons. The acrylic sphere, PMTs and PMT support structure were re-used from the original Sudbury Neutrino Observatory (SNO) experiment. As a result, SNO+ strongly resembles its predecessor SNO with an updated feature, a new rope-net, to hold the vessel in place as it becomes buoyant when filled with liquid scintillator.
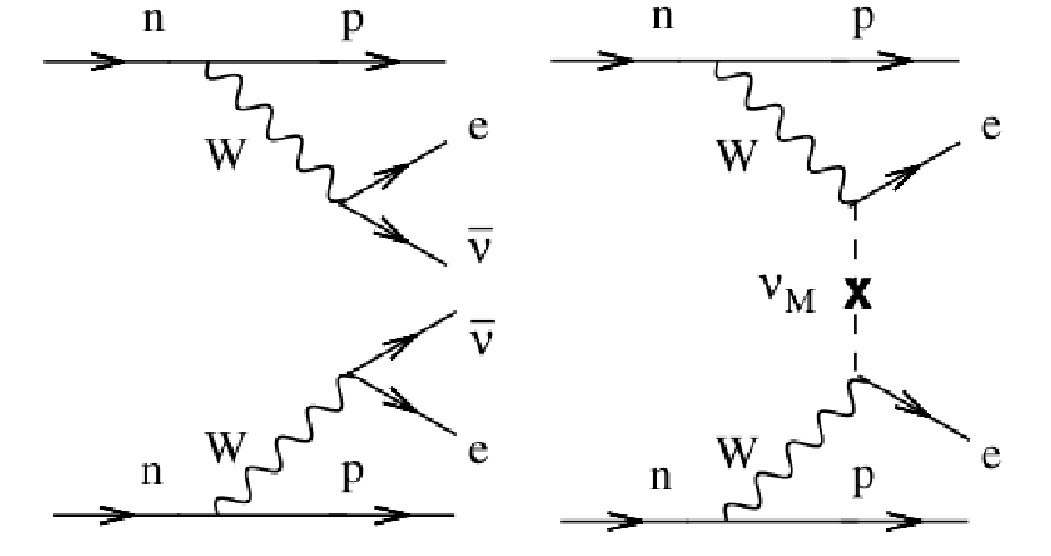
The liquid scintillator used in the SNO+ experiment will be primarily composed of linear alkylbenzene (LAB), which was first identified by Queen’s and BNL for this type of experiment. LAB has good light output, good optical properties, is a "mild" chemical to work with (it has properties much like those of mineral oil) and is compatible with the SNO+ acrylic vessel. LAB is the base chemical to manufacture dish soap, among other things, which means that it is commercially available and non-toxic for chemical safety. The CEPSA Química plant in Bécancour, Québec produces excellent LAB quality for SNO+.
One of the many advantages of using LAB as liquid scintillator is the possibility to dissolve heavy metals such as tellurium with long term stability and good optical properties. SNO+ searches for neutrinoless double-beta decay (0νββ) in tellurium (130Te). 0νββ-decay is a rare nuclear process that may happen if neutrinos are their own antiparticles. Understanding the Majorana nature of neutrinos is one of the most active areas of research in modern neutrino physics. The observation of the 0νββ-decay would demonstrate lepton number violation, a key ingredient in the theory of leptogenesis.
Images Courtesy: SNO+ Experiment Website
Daya Bay Reactor Antineutrino Experiment
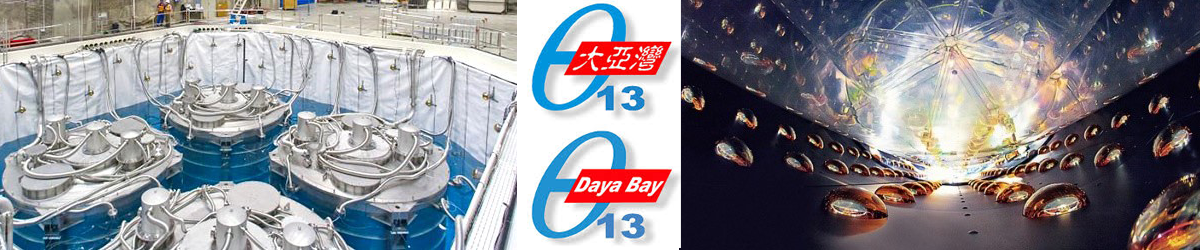
The Daya Bay Neutrino Experiment is a neutrino-oscillation experiment designed to measure the mixing angle θ13 using antineutrinos produced by the reactors of the Daya Bay Nuclear Power Plant (NPP) and the Ling Ao NPP. Recent discoveries have shown that neutrinos are not massless. Mixing among the mass states has been observed in atmospheric and solar neutrinos in experiments such as Super-K and SNO as well as in experiments using manmade neutrino sources including KamLAND and K2K.
The goal of the Daya Bay experiment is a measurement of sin22θ13 to 0.01 or better, an order of magnitude better sensitivity than limit achieved by CHOOZ. The experiment measures the flux of antineutrinos from the reactors via the inverse beta-decay reaction, and any deficit from the expected 1/L2 dependence is a signature for neutrino oscillations. Inverse beta-decay consists of the capture of an electron antineutrino on a proton (hydrogen) resulting in the production of a positron and a neutron. The number of inverse beta-decay reactions is determined by counting the coincidence of the energy deposited by the positron followed by the energy released from the neutron capture on hydrogen (~2.2 MeV) ~200 µs or gadolinium (~8 MeV) ~30 µs later. The most serious backgrounds are from cosmic rays. The reactor complex near Daya Bay, China, about 70 km northeast of the Hong Kong airport, is an excellent site for the experiment, and one of the few appropriate sites worldwide. Accomplishing the experiment requires powerful reactors in a geological setting that allows for large underground neutrino detectors with significant overburden.
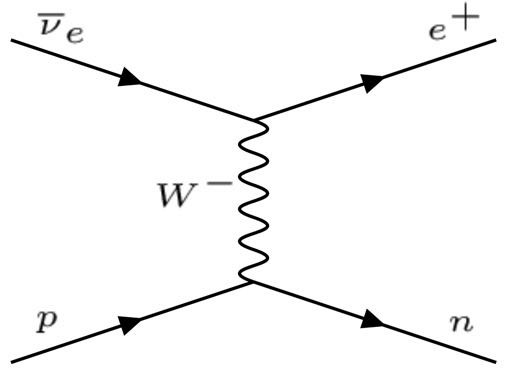
Antineutrinos are detected via the inverse beta-decay (IBD) reaction, ����̅e + p → e+ + n, in a delayed coincidence between the prompt signal of the positron and the subsequent capture of the neutron in a (n,g) reaction after it has been thermalized in the scintillator. The neutron capture can occur on hydrogen in the liquid scintillator (LS). The cross-section is 0.332 barns and the energy of the emitted gamma is 2.2 MeV. There are several important advantages of adding a neutron-capture-enhancing element, such as gadolinium (Gd), to the LS. The (n,g) cross-section for natural Gd is 49,000 barns (with major contributions from the 155,157Gd isotopes), that only a small concentration of Gd, e.g. 0.1% by mass, is necessary. The neutron-capture on Gd releases 8-MeV of energy in a cascade of 3-4 g-rays that can easily exclude the low-energy backgrounds from natural radiations in the surrounding environment.
The precision of the measurements at Daya Bay is improved by using a "near" and "far" detector configuration with multiple detector modules (4 suites) to increase signal statistics and to suppress the systematic uncertainties related to the anti-neutrino flux from the reactor. The Daya Bay detector system is composed of eight functionally identical antineutrino detectors (ADs) located in near and far underground halls close to the six reactor cores. Each AD has three liquid zones: gadolinium-loaded liquid scintillator (Gd-LS, neutrino target), unloaded liquid scintillator (LS, g-catcher), and mineral oil (MO, shielding buffer) situated in the order of inner- to outer-most.
The Daya Bay Gd-LS consists of linear alkylbenzene (LAB), as the detection medium. 3,5,5-trimethylhexanoic acid (TMHA) is chosen as the ligand because its Gd-complex has good stability in LAB and is easy to produce in large quantities. The Daya Bay Gd-LS also consists of 3 g/L 2,5-diphenyloxazole (PPO) as the fluor and 15 mg/L p-bis-(o-methylstyryl)-benzene (bis-MSB) as the wavelength shifter. After years of development, a production scheme for the fabrication of a Gd-complex solid, followed by direct dissolution in scintillator was chosen to accommodate the logistics and operation at the Daya Bay nuclear power plant, and to produce stable Gd-LS for the Daya Bay experiment.
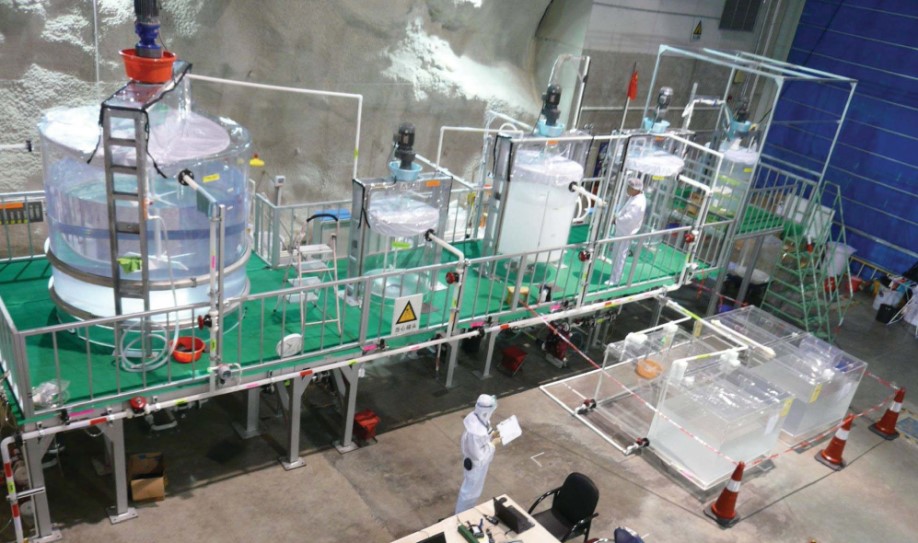
In 2012 the Daya Bay collaboration announced the discovery that θ13 ≠ 0 with a statistical significance of 5.2σ. The campaign to measure the mixing angle as precisely as possible has not stopped. The most precise measurement of θ13 = 0.084 ± 0.005 was published in 2015 at the Moriond physics conference and represent the represents the best fit for the mixing angle and mass difference.
Images Courtesy: Daya Bay Experiment Website
PROSPECT (Precision Oscillation and Spectrum Experiment)
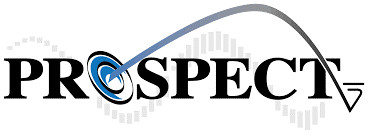
PROSPECT is a reactor neutrino experiment at very short baselines to make a precision measurement of the flux and energy spectrum of antineutrinos emitted from nuclear reactors. PROSPECT will search for the oscillation signature of sterile neutrinos and test our understanding of the emission of antineutrinos from the fission products in a nuclear reactor. The measurements of PROSPECT will test our understanding of the Standard Model of Particle Physics, deepen our understanding of nuclear processes in a reactor, and help develop technology for the remote monitoring of nuclear reactors for safeguard and non-proliferation.
A recent analysis of previous reactor neutrino experiments, including Daya Bay, suggests a deficit in the observed number of reactor antineutrinos compared to expectations. Such a deficit could be due to the existence of a new, yet undetected particle (a sterile neutrino) or point to a lack of understanding in the modeling of neutrino emission from nuclear reactors. Recent experimental results have also shown that the energy distribution of antineutrinos from nuclear reactors may be different than expected. PROSPECT, a precision oscillation and spectrum experiment, located at the High Flux Isotope Reactor (HFIR) at ORNL will measure the antineutrinos from HFIR at a distance of less than 10m to resolve these questions. In addition, a successful measurement of reactor antineutrinos with a detector in or near the HFIR will open new ways for nuclear safeguarding and reactor monitoring.
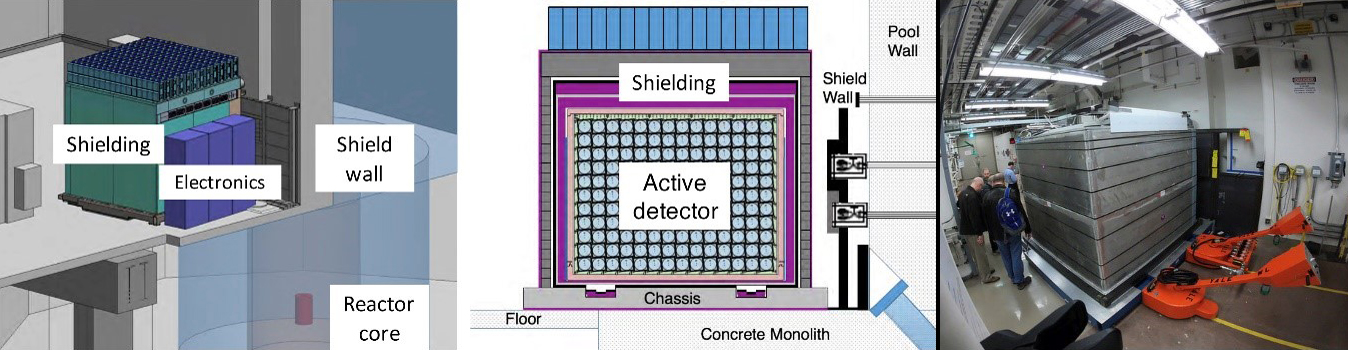
The detectors consist of an optically segmented antineutrino target of 6Li-doped liquid scintillator surrounded by external passive shielding. Light produced in antineutrino interactions is efficiently propagated using highly specular reflecting segment walls and collected at each segment’s end with a photomultiplier tube. The PROSPECT detector (AD) required a liquid scintillator (LS) with both very good PSD for background rejection of fast neutron and ambient γ-ray background (i.e. better than the linear alkyl benzene used in Daya Bay experiment) and high light yield for energy resolution. The compactness of the AD, as well as the length-scale of the segmentation, strongly preferred doping with a neutron capture agent yielding only charged particles and thus a topologically compact capture signature. Furthermore, a low-toxic, non-flammable formulation was needed to support ease of deployment within the HFIR reactor building.
To meet these requirements, the PROSPECT collaboration developed a novel lithium-doped liquid scintillator (Li-doped LS) formulation based on commercially available chemicals. The Li-doped LS was manufactured in house by our group, at the Brookhaven National Laboratory (BNL). Li-doped LS consists of a mix of complexing agents, 10 mol/L aqueous 6Li chloride, 2,5-diphenyloxazole (PPO) as the fluor, and 1,4-bis (2-methylstyryl) benzene (bis-MSB) as the wavelength shifter in a commercial, di-isopropylnapthalene (DIN)-based scintillator (EJ-309). The formula was developed based on principal of Water-based Liquid Scintillator (WbLS link to research/detector page).
Images Courtesy: PROSPECT Collaboration
LZ Dark Matter Experiment
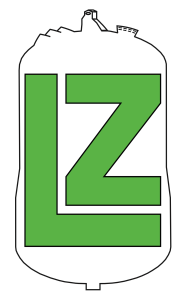
LZ is a next-generation dark matter direct detection experiment. LZ has been selected to be one of three funded G2 dark matter experiments. The LZ collaboration consists of 250 scientists and engineers from 37 institutions in the U.S., U.K, Portugal, Russia and Korea.
The LZ detector will operate at the Sanford Underground Research Facility (SURF) in Lead, South Dakota. SURF is located at the former Homestake gold mine and has laboratory spaces underground. LZ will be installed in the Davis Laboratory 4,850 ft underground (nearly a mile). This is the same site that housed Ray Davis’ Nobel Prize-winning neutrino experiment. The LZ detector consists of 7 tonnes of liquified xenon to detect faint interactions between galactic dark matter and regular matter. Dark matter comprises about 85% of the mass of the Universe, and its particle nature is still unknown.
The direct detection of dark matter in earthbound experiments depends on the local properties of the Milky Way’s dark matter and the properties of the dark matter particles themselves. The local properties of the Milky Way’s dark halo are determined by astrophysical studies and include the local dark matter mass density as well as the distribution of the velocities of dark matter particles. The conjecture that the dark matter particles are weakly interacting massive particle (WIMP) implies that their scattering with nuclei is non-relativistic two-body scattering; in LZ, we seek to observe the xenon nuclei that recoil after having been struck by an incoming WIMP. The mass assumed for the WIMP determines the kinematics of the scattering, and the rate of WIMP-nucleus scatters seen in a WIMP detector further depends on the exposure defined as the product of the target mass and the live time, the WIMP-nucleus cross-section, and the energy threshold for detection of the nuclear recoil.
The core of the LZ experiment is a two-phase xenon (Xe) time projection chamber (TPC) containing 7 fully active tons of LXe. Scattering events in LXe create both a prompt scintillation signal (S1) and free electrons. Electric fields are employed to drift the electrons to the liquid surface, extract them into the gas phase above, and accelerate them to create a proportional scintillation signal (S2). Both signals, S1 and S2, are detected by arrays of photomultiplier tubes (PMTs) above and below the central region. The difference in time of arrival between the signals measures the position of the event in z, while the x, y position is determined from the pattern of S2 light in the top PMT array. Events with an S2 signal but no S1 are also recorded. The heart of the LZ detector (including the inner titanium cryostat) will be assembled on the surface at SURF, lowered in the Yates shaft to the 4850L of SURF, and deployed in the existing water tank in the Davis Cavern.
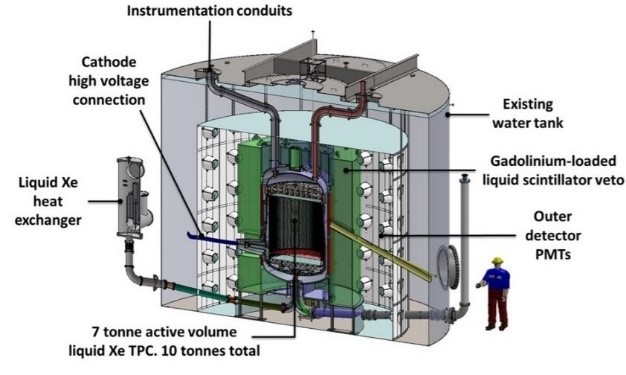
The LZ detector includes several added capabilities beyond the successfully demonstrated LUX and ZEPLIN designs. The most important addition is a nearly hermetic liquid organic scintillator (ultrapure gadolinium loaded liquid scintillator, Gdm-doped LS) outer detector, which surrounds the central cryostat vessels and the TPC. The Gdm-doped LS have the same formula as that used for the Daya Bay detectors, but with superior radiopurity, and is produced here by the NNC at BNL. The outer detector and the active Xe “skin” layer, the Xe between the inner cryostat wall and the outer wall of the TPC, operate as an integrated veto system, which has several benefits. The first is rejecting gammas and neutrons generated internally (e.g., in the PMTs) that scatter a single time in the fully active region of the TPC and would otherwise escape without detection; this could mimic a WIMP signal. As these internally generated backgrounds interact primarily at the outer regions of the detector, the veto thus allows an increase in the fiducial volume.
Images Courtesy: LZ Dark Matter Collaboration
AIT/NEO
AIT (Advanced Instrumentation Testbed)/NEO (Neutrino Experiment One), a transformation from WATCHMAN (WATer CHerenkov Monitor for AntiNeutrinos), is a collaborative United States–United Kingdom effort to pursue a research and development program based on a multi-purpose neutrino detection platform. The overall vision of AIT/NEO is to take advantage of synergies between pure and applied neutrino research by studying neutrinos emitted by reactors, the Earth, the Sun, and the distant stars–aiming for nuclear security and fundamental science applications.
Once constructed, the first part of AIT/NEO will consist of a large underground cavern and associated laboratory space. The AIT will permit deployment of numerous advanced technologies that are relevant for remote reactor monitoring and detection, including water-based liquid scintillators, fast photo-sensors, light concentrators, and others. AIT/NEO plans to operate 1.1 kilometers underground at the site of the Boulby Underground Laboratory—an existing U.K. government-funded deep underground science facility operating in a working potash, polyhalite, and salt mine (Boulby Mine) located on the northeast coast of England—and about 25 kilometers away from the Hartlepool nuclear power station. The Hartlepool station emits large numbers of antineutrinos, providing AIT/NEO with a convenient and free source for the exploration and development of antineutrino detection capabilities.
The primary goal of the AIT/NEO-WATCHMAN is to detect reactor antineutrinos using a large water-based detector and thereby observe reactor operations at a significant distance from a nuclear reactor complex. The NEO detector—the first to be installed at AIT—will consist of approximately 5000 tons of highly purified liquid. Doping the water with trace amounts of gadolinium (Gd-H2O) to improve neutron capture signal, or organic scintillator (WbLS, link to research/detector page) to allow low-energy threshold, is expected to significantly enhance its sensitivity to antineutrinos. Due to its relatively low cost and attractive scaling properties, Demonstration of this water-based detection technique is a key step forward in the long-term goal of building large detectors with greater standoff sensitivity to the existence and operational conditions of nuclear reactors. AIT/NEO will explore the potential of antineutrino detection systems to monitor man-made nuclear reactors on Earth to assist international efforts to track nuclear-weapons-usable materials, and thereby restrict the growth and spread of nuclear weapons and weapons development around the world. In addition, AIT/NEO will join the ranks of a handful of large, high-sensitivity astrophysics research detectors built worldwide seeking to detect neutrino emission from nearby exploding stars (supernovae). Observation and analysis of supernova neutrino bursts provides insight into stellar growth and collapse, the formation of elements in the universe with atomic masses greater than that of helium, and the mysterious properties of neutrinos themselves.
The potential detector medium, water based liquid scintillator, for AIT/NEO is currently being developed by the NNC group at BNL. The successful deployment of WbLS at AIT/NEO will be the world first hybrid Cerenkov and Scintillation detector for the scientific community.
Results: First data-taking aims in 2026.
THEIA
THEIA is a future large-scale multi-purpose detector, aiming to utilize water-based liquid scintillator (WbLS) or lightly doped oil as its detector medium coupled with very fast timing, large area picosecond photon detectors (LAPPD) for nuclear and particle physics frontiers. The main prospects of THEIA experiment include, but are not limited to, CP violation in the lepton sector, natures of neutrino as Majorana particle and its mass, along with solar and supernovae neutrino detection using loading of different isotopes in the detector medium.
The development of THEIA is still at very early design stage with varied working groups consisting of many international collaborators for technical developments.
Past Experiments
SNO
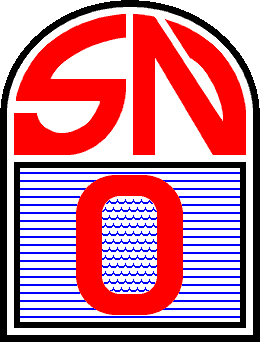
The Sudbury Neutrino Observatory (SNO) was an experiment that was carried out by an international scientific collaboration with data collected during the period from 1999 to 2006. By using heavy water as a detection medium, the SNO experiment demonstrated clearly that solar electron neutrinos from 8B decay in the solar core change into other active neutrino flavors in transit to Earth. The reaction on deuterium that has equal sensitivity to all active neutrino flavors also provides a very accurate measure of the initial solar flux for comparison with solar models.
The Sudbury Neutrino Observatory (SNO) was initiated in 1984 primarily to provide a definitive answer to the Solar Neutrino Problem. Ever since the pioneering calculations of solar neutrino fluxes by John Bahcall and the pioneering measurements by Ray Davis in the 1960s, it was known that there was a discrepancy between the observed fluxes and the calculations. It was determined that a detector filled with heavy water could be used to perform a sensitive measurement and determine whether the neutrinos change their type in transit from the core of the Sun. The unique properties of deuterium could make it possible to observe both the electron neutrinos produced in the core of the Sun and the sum of all neutrino types. The SNO experiment was able to measure two separate reactions on deuteron (d):
νe + d → p + p + e−
a charged current (CC) reaction that was sensitive only to electron-flavor neutrinos, and
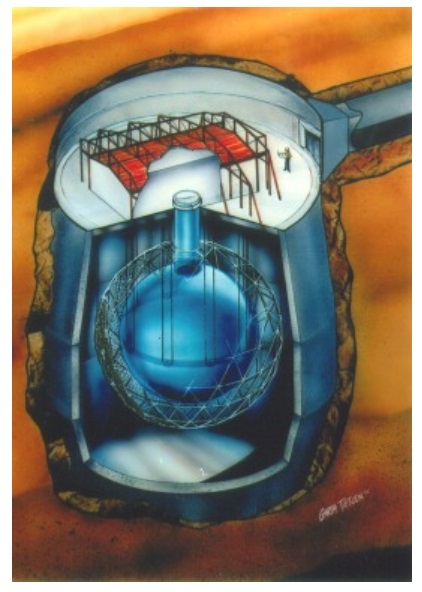
νx + d → n + p + νx
a neutral current (NC) reaction that was equally sensitive to all neutrino types. In addition, the SNO detector could observe neutrinos of all flavors via the elastic scattering (ES) of electrons by neutrinos:
νx + e− → νx + e−
which is six times more sensitive to electron neutrinos than other flavors.
The cavity was 34 meters high by 22 meters in diameter at the equator, lined with a water- and radon-impermeable Urylon plastic. The detector was situated 2 km underground in an active nickel mine owned by Vale (formerly Inco Ltd) near Sudbury, Ontario. The central element was 1000 tonnes of heavy water (>99.5% isotopically pure), on loan from Atomic Energy fo Canada Limited (AECL) and housed in a transparent acrylic vessel (AV) 12 meters in diameter and 5 cm thick.
The value of the heavy water was about $300 million Canadian dollars. The heavy water was viewed by 9438 20-cm diameter Hamamatsu R1408 photomultiplier tubes (PMT) mounted on a stainless-steel geodesic photomultiplier support frame (PSUP). Each PMT had a 27-cm entrance light concentrator to increase the effective photocathode coverage to 54%. A further 91 PMTs without concentrators were mounted looking outward from the PSUP to observe events entering the detector from the outside. The entire cavity outside the acrylic vessel was filled with 7000 tonnes of ultra-pure ordinary water for additional shielding from background radiation.
Images Courtesy: SNO Collaboration
LENS
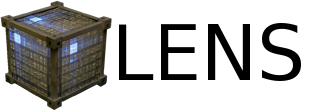
The goal of the LENS experiment is to measure, in real time, the primary low energy (sub-MeV) solar neutrino spectrum, with special attention to the pp-, CNO- and 7Be-neutrinos. The detector is an indium based liquid scintillator.
The main reaction is:
νe + 115In → e- (Ee- = Eν - 115keV) + 2 delayed γ's (coincidence tag) + 115Sn
The interaction of a neutrino with the target nucleus is followed by a coincident γ-emission providing a powerful signature of νe interactions compared to background events. LENS will determine the spectral shape of the low energy solar neutrino spectrum for the first time. Up until now we have only measured spectral shape of the higher energy 8B portions of the spectrum (~5% of the total spectrum). The low-energy part of the neutrino spectrum is very important, since the Sun's energy is primarily produced in the pp-chain, which produces neutrinos only in the low energy portion of the spectrum. Measuring these neutrinos probes the dominant energy production mechanism in the sun. With new data on the low energy band of the spectrum we can answer questions such as: Is the Sun getting hotter? Are there sub-dominant non-nuclear sources of energy in the Sun?
The LENS design is a cubic lattice of low index of refraction material (Teflon FEP) in a higher index of refraction liquid (Indium liquid scintillator (InLS)). The cubic lattice structure of LENS allows for precision digitized localization of events in the detector allowing for extraordinary background reductions.
Image Courtesy: LENS Collaboration