Quark Matter 2018: Nuclear Physicists Gather to Discuss Fundamental Particle Interactions
May 11, 2018
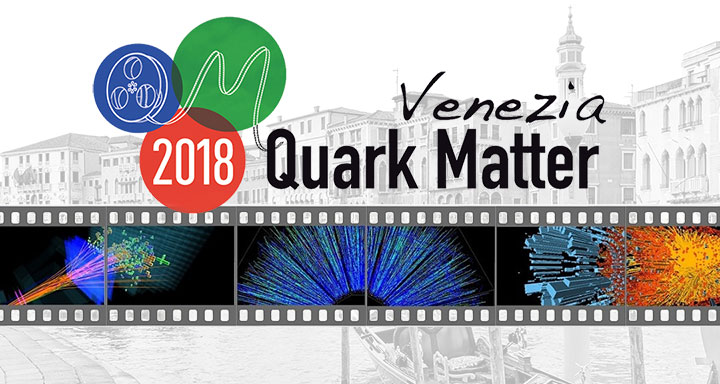
Banner of the Quark Matter 2018 meeting website (Credit: Italy's National Institute for Nuclear Physics, INFN).
UPTON, NY—Nuclear physicists from around the world seeking to understand the intricate details of the building blocks of visible matter are meeting in Venice, Italy, May 13-19, to discuss the latest results and theoretical interpretations of data from the world’s premiere collider facilities.
High-energy collisions of atomic nuclei at the Relativistic Heavy Ion Collider (RHIC) at the U.S. Department of Energy’s (DOE) Brookhaven National Laboratory and the European Large Hadron Collider (LHC) briefly liberate the fundamental particles that make up protons and neutrons—known as quarks and gluons—from their confinement within these particles so nuclear physicists can study them as they existed at the dawn of the universe. Physicists also explore the “quark-gluon plasma” created in these collisions to learn about the strong nuclear force—the strongest force in Nature—which holds quarks and gluons together in the ordinary matter of everything we see in the universe today.
This will be the 27th International Conference on Ultra-Relativistic Nucleus-Nucleus Collisions—more commonly referred to as Quark Matter 2018—and the 13th since RHIC, a DOE Office of Science user facility for nuclear physics research, began operations in 2000.
“These Quark Matter meetings started with the discussion of earlier experiments that in many ways guided the scientific goals and construction of RHIC and the plan to have a heavy-ion physics program at the LHC,” said James Dunlop, Associate Chair for Nuclear Physics in Brookhaven Lab’s Physics Department and a researcher on RHIC’s STAR experiment. “At each successive conference, physicists in this field present data collected with ever-higher precision and use comparisons with theory to refine explanations for what we are observing.”
“The fact that RHIC and the LHC can measure things very similarly with similar precision in very different systems—in terms of the temperature and density created in the collisions—means we can learn much more than we would by measuring at just one point,” Dunlop said.
Most talks are focused on particle interactions, but the meeting also offers attendees—including students just entering the field—the chance to interact.
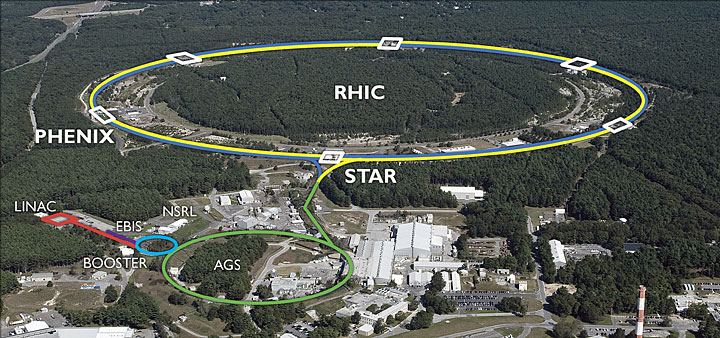
This aerial view of the Collider Accelerator complex at Brookhaven National Laboratory shows the locations of the STAR and PHENIX detectors at regions where the two rings of the Relativistic Heavy Ion Collider (RHIC) cross to allow particle interactions.
“Getting everybody together, mixing students with senior faculty and scientists across the various experimental collaborations, research facilities, and theorists—not just within their own groups—gives people ideas of what to look at next,” said Helen Caines, a Yale University physicist who is a co-spokesperson for the STAR collaboration at RHIC.
Several sessions will be devoted to exploring possible future physics facilities, including a proposed U.S.-based Electron-Ion Collider (EIC). At an EIC, physicists hope to explore how the arrangement and interactions among quarks and gluons within protons and atomic nuclei establish the fundamental properties of those particles and the ordinary nuclear matter within and around us.
Highlights of Presentations from RHIC
RHIC currently hosts two experimental collaborations, each with a complex particle detector for tracking and analyzing collision data—STAR, which is still operating, and PHENIX, which is undergoing a major transformation into a new detector known as sPHENIX, but still has ample data to analyze from experimental runs prior to 2018. At Quark Matter 2018, these collaborations will present high-precision data on details of the “perfect liquid” quark-gluon plasma (QGP) created when two heavy ions such as gold nuclei collide, the characteristics needed to create a system of this early-universe substance, and comparisons with results from a range of other collision systems, including proton-proton, proton-gold, and different types of nuclei.
Differences among heavy particles
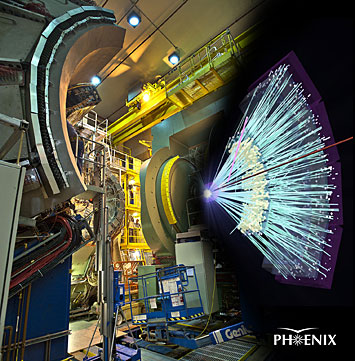
Split-screen view of the PHENIX detector at Brookhaven National Laboratory's Relativistic Heavy Ion Collider (RHIC) and particle tracks recorded by the detector in a collision of two gold nuclei.
Since RHIC’s earliest days, scientists have studied the collective behavior of particles emerging from the collisions. They’ve interpreted certain patterns of particle flow observed in the high-energy gold-gold collisions as evidence of the liquid-like properties of the QGP. Building on data presented at the last Quark Matter meeting in February 2017, PHENIX will present data that look in detail at how different types of heavy particles get caught up in this flow. With four times as much data, PHENIX will now be able to disentangle signals from so-called charm and even heavier bottom quarks and present the first results on how each of these flow.
PHENIX will also present data on correlations in the way pairs of particles called muons emerge with respect to one another perpendicular to the path of the colliding beams in proton-proton collisions. “This analysis provides a picture of how the heavy quarks are produced from the underlying quark and gluon interactions,” said Stefan Bathe, a professor at Baruch College, City University of New York, and a deputy spokesperson for PHENIX. The PHENIX data indicate that this process turns out to be different for charm and bottom quarks, contrary to observations at higher energy at the LHC.
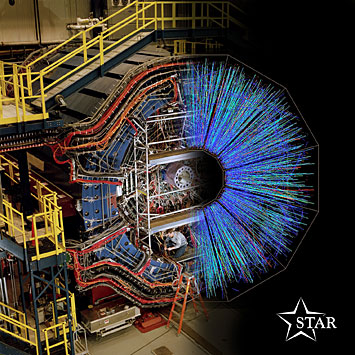
Split-screen view of the STAR detector at Brookhaven National Laboratory's Relativistic Heavy Ion Collider (RHIC) and particle tracks recorded by the detector in a collision of two gold nuclei.
STAR will also address questions of how different types of heavy particles behave. For example, they will present a new analysis of results from the high-precision “Heavy Flavor Tracker” (HFT) that show how one type of heavy-quark-containing particle known as a D-zero meson moves out of the plasma. The results reveal that these heavy particles have a smaller “radial velocity” than particles made of light quarks. That means these heavy particles “freeze out”—become composite particles and stop interacting with the plasma—sooner than composite particles made of lighter quarks.
“These results give us snapshots at different times in a system that lasts only a miniscule fraction of a second,” said Caines. “We can use them to disentangle what happens when—what part of a particle’s behavior is due to what happens in the quark-gluon plasma and what happens later—to learn more about the plasma.
“It’s kind of like, if you watch someone running in a race and you only measure her speed at the start and the end, you only have rather limited information. But if you can measure at different points, you may be able to get some idea about what happened during the race, for instance did she run at a steady pace or stop and take a break in the middle.”
STAR will also present data comparing how composite particles containing heavy charm quarks form in gold-gold collisions and in proton-proton collisions. It appears that these charm quarks get incorporated into different types of composite particles in the two systems.
“If you have a bath of many quarks and gluons, as you do in the gold-gold collisions, it appears to be easier to make exotic types of particles than if you have a smaller bath [in proton-proton collisions],” Caines said. “This adds to our evidence that something different is happening in these two systems,” she noted, explaining that the gold-gold collisions routinely create quark-gluon plasma while the proton-proton collisions generally do not.
QGP in small systems
There is, however, a growing body of evidence that QGP can be created where scientists least expected it. PHENIX will present extensive data analyzing collisions of relatively small particles (protons, deuterons, and helium-3 nuclei, made of one, two, and three particles, respectively) with gold ions. Earlier data hinted that these collisions appear to create tiny drops of QGP at the point of impact because they all produce particle flow patterns that are remarkably similar to those observed in high-energy gold-gold collisions, albeit on a smaller scale. Intriguingly, the deuteron-gold collisions produced mostly elliptical droplets, while the droplets produced in helium-gold collisions emerged primarily in triangular patterns.
To confirm if these flow patterns were indeed triggered by the formation of small drops of QGP, PHENIX explored whether the flow patterns mimic the shape of the projectile penetrating the gold nucleus, as would be expected when a near-perfect fluid is formed. There was also a possibility that these shaped flow patterns could have been caused, instead, by an initial-state interaction among the particles without the formation of QGP.
“We have now completed measurements of the strength of the triangular and elliptic flow patterns in three different systems with very different initial geometries,” said Julia Velkovska, a deputy spokesperson for PHENIX from Vanderbilt University. “These measurements, taken together, rule out the possibility that the flow patterns are triggered mainly by initial-state effects. Models that include QGP formation, on the other hand, were able to predict the results observed in all three systems in a very clear and quantitative way, strengthening our confidence that these flow patterns are triggered by the formation of QGP.”
A possible look at initial state conditions
A similar connection between shape and flow was apparent in the earliest measurements of flow at RHIC, where the elliptical flow pattern observed in high energy gold-gold collisions was linked to the elliptic shape formed by two partially overlapping gold ions at the earliest stage of a collision (picture two spheres colliding off-center to create a football-shaped overlap region). RHIC scientists assumed that the orientation of this elliptic shape would be the same at different longitudinal points along the path of the colliding ions. But now, STAR has new results from measurements at different longitudinal points that suggest a significant twist of the initial ellipse along this path.
“In order for these details of the initial state to survive and leave an imprint on the transverse flow pattern in the final state, the quarks and gluons in the interacting ions must collide very frequently,” said Caines. “These new measurements are consistent with previous findings that the matter created in the collisions behaves as a nearly perfect liquid with very low shear viscosity, or resistance to flow.”
In principle, these measurements might allow scientists to discern among different theoretical descriptions of how energy is distributed in the transverse and longitudinal direction right after the collision. “But what we see is a much stronger signal than any of the models predict,” said Caines. “We have a hint that we don’t understand, so we want to keep looking at what this initial state looks like. No matter what, these new data provide important new clues about the 3D structure of the initial state."
PHENIX has also made strides in deciphering the initial state. At Quark Matter, the collaboration will present new measurements of elliptic and triangular flow patterns from gold-gold collisions with different degrees of centrality, or overlap between the colliding spherical nuclei. These new measurements were performed on an event-by-event basis, instead of determining the average flow strength over many collisions. They contain essential information about the fluctuations in the initial state and how the near-perfect fluid translates those to the final-state particle distributions. The collaboration also made complementary measurements based on multi-particle correlations. “We are now in a position to determine details of the flow patterns that can distinguish between initial-state fluctuations and additional sources of fluctuations during the evolution of the QGP,” Velkovska said.
Using photons to track the Quark-Gluon Plasma transition
PHENIX will present new data on photons—particles of light—emitted from a wide range of heavy ion collision systems, including gold-gold and copper-copper, and compare these data to those in lead-lead collisions at the LHC.
The color of the photons emitted by the matter created in heavy-ion collisions carries information about the temperature—think of a piece of iron glowing red in a blacksmith’s fire, or an even hotter white glow. PHENIX has used such measurements of “direct photons” to establish that RHIC’s gold-gold collisions create temperatures more than hot enough to create quark-gluon plasma.
The newer measurements compare the rate of photon production in the various heavy-ion systems and also in proton-proton collisions. PHENIX discovered a common behavior of photon production in heavy ion collisions that is different from photon production in proton-proton collisions.
“That means all heavy-ion systems, independent of energy and system size, have something in common but are different from proton-proton systems,” Bathe said.
But what about those collisions of small particles with heavy ions? “If there are small drops of QGP in small systems, there should also be thermal photons emitted from those collisions,” Bathe said. “We will present data from proton-gold and deuteron-gold collisions that show that those small systems bridge the transition from proton-proton to heavy ion collisions.”
Swirliest matter redux
STAR will also present much more detail about the record-setting vorticity, or swirliness, of the QGP, following up on a paper published last summer that revealed how they track this swirling by measuring the spin alignment of certain particles emitted from the plasma. The new analyses are based on 150 times more data. They include comparisons of how vorticity varies depending on how central, or head-on, the collisions are. The closer to head-on the collisions get, the lower the vorticity, which is what the scientists expected since it’s the off-center collisions that set the QGP swirling. More data being collected in this year’s RHIC run should allow the physicists to use these measurements to determine the strength of the QGP’s magnetic field.
Research at RHIC is funded primarily by the DOE Office of Science, and also by these agencies and organizations.
Brookhaven National Laboratory is supported by the Office of Science of the U.S. Department of Energy. The Office of Science is the single largest supporter of basic research in the physical sciences in the United States, and is working to address some of the most pressing challenges of our time. For more information, please visit science.energy.gov.
Follow @BrookhavenLab on Twitter or find us on Facebook.
2018-12847 | INT/EXT | Newsroom