Observing Oxidation at the Atomic Level with Guangwen Zhou
interview with a CFN user
September 15, 2021
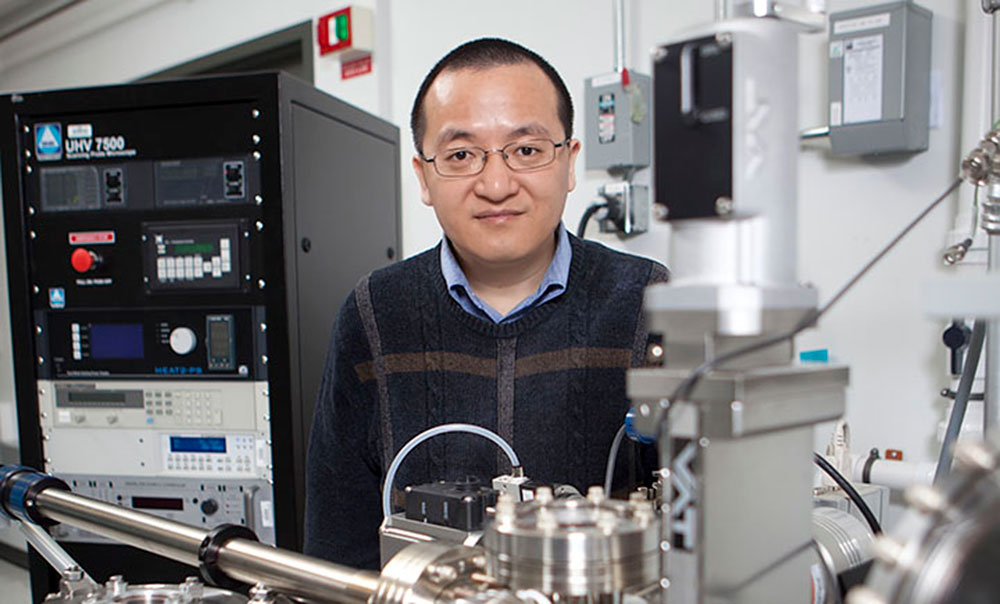
Professor Guangwen Zhou in the Surface and Interface Laboratory at Binghamton University. Credit: Binghamton University.
For more than 100 years, scientists have studied chemical reactions in which elements combine with oxygen to form oxides. Traditionally, studies on oxides relied on ex situ characterization, which captures changes after a reaction has occurred. But today, instruments capable of monitoring dynamic processes as they happen in real time and under reaction conditions are providing new insights into oxidation at the atomic scale. Several of these in situ and operando instruments exist at the Center for Functional Nanomaterials (CFN), a U.S. Department of Energy (DOE) Office of Science User Facility at Brookhaven National Laboratory. Since 2010, Guangwen Zhou—a professor in the Mechanical Engineering Department, interim director of the Materials Science and Engineering Program, associator director for the Institute for Materials Research, and principal investigator of the Surface and Interface Laboratory at Binghamton University, State University of New York (SUNY)—has been performing in situ and operando microscopy and spectroscopy in the CFN Electron Microscopy, Proximal Probes, and Theory and Computation Facilities to understand the fundamental mechanisms of oxidation. Prior to joining Binghamton faculty in 2007, Zhou was a research assistant professor at the University of Pittsburgh, postdoctoral researcher at Argonne National Laboratory, and research associate at Beijing Laboratory of Electron Microscopy at the Chinese Academy of Sciences. He received a PhD in materials science from the University of Pittsburgh, a master’s degree in physics from Beijing University of Technology, and a bachelor’s degree in physics from Xiangtan University. Over his career, Zhou has published more than 200 journal papers and received several awards, including the National Science Foundation Faculty Early Career Development Program (CAREER) Award and the SUNY Chancellor’s Award for Excellence in Scholarship and Creative Activities.
The Surface and Interface Laboratory at Binghamton “seeks to understand the atomic processes of a variety of surface and interface reactions.” Which atomic processes and reactions does your lab focus on?
Oxidation is the common thread connecting different projects within my lab. We study two kinds of reactions: those occurring at surfaces and those at interfaces. For the surface reactions, we look at how oxygen molecules attack metal surfaces to form surface oxides. This type of reaction between gas molecules and solid surfaces shares several similarities with catalytic reactions. So, my group does some work related to catalysis, too. For the interface reactions, we look at what happens at the interface between the oxide coating and metal surface. Surfaces and interfaces are where chemistry happens. We need to understand what is going on at an atomic level in both places to control oxidation from the very early to latter stages.
Why would you want to control oxidation?
Oxidation leads to the corrosion of metallic materials. Corrosion severely limits the lifetime of these materials. Perhaps the most familiar example of corrosion is iron coming into contact with oxygen in the air to form iron oxide, more commonly known as rust. An estimated three percent of the U.S. gross domestic product goes toward the repair of damage caused by corrosion—for example, to water pipes.
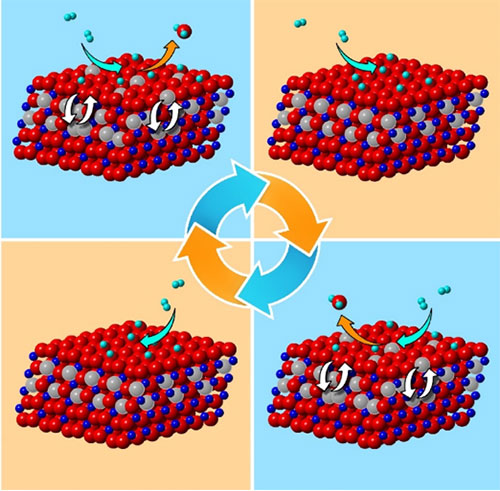
Using ambient-pressure x-ray photoelectron spectroscopy at the CFN, Zhou and his team captured the chemical process along with atomic-scale structural changes of the surface and subsurface of copper oxide catalysts for hydrogen oxidation in real time. This illustration shows water molecules forming at the surface while oxygen vacancies (spots where oxygen is missing) in the subsurface order and disorder. This ordering and disordering results in structural oscillations in deeper atomic layers. Research published in Nature Communications 11, 305 (2020).
Oxidation is not only related to corrosion. As I mentioned, oxidation occurs in many types of catalytic reactions. A lot of catalysts are based on metallic materials, and the reactions often involve oxygen. For example, one catalytic reaction we study in my group is carbon monoxide oxidation, converting carbon monoxide (a poisonous gas produced in automobile exhaust) into carbon dioxide. Another is methanol oxidation, an important reaction for hydrogen production and energy source for fuel cells. We’re also doing some studies on oxidation of hydrogen to produce water.
How does your group study oxidation at the atomic level?
You cannot develop a full understanding of oxidation based on a single tool. We use a variety of surface-science characterization tools to probe the reaction from different angles. In my lab at Binghamton, we have a system that combines scanning tunneling microscopy, atomic force microscopy, electron spectroscopy, and low-energy electron diffraction. We also have access to electron microscopes and surface analysis instruments through Binghamton’s Analytical and Diagnostics Laboratory. We use these on-campus facilities to perform preliminary experiments and provide hands-on training to students on how to run the experiments. At the CFN, my students and I have access to more advanced and unique capabilities—especially in situ ones—that don’t exist at Binghamton.
What are some of the advanced and unique capabilities you leverage at the CFN?
One instrument we regularly use is the in situ environmental transmission electron microscope (TEM). With this specialized TEM, we can watch how oxidation happens in real time as we simultaneously heat our sample and introduce reactive gases like oxygen and water vapor. The videos generated by the TEM have very high resolution in both space and time. This high resolution allows us to monitor the reaction atom by atom to see any structural changes. The microscope is equipped with an ultrafast camera, which can take 400 image frames per second, allowing us to capture transient phenomena or intermediate states in the reaction.
Another capability is x-ray photoelectron spectroscopy (XPS). In the traditional XPS setup, scientists look at samples under ultrahigh vacuum conditions. But the XPS tools at the CFN and National Synchrotron Light Source II (NSLS-II) can operate at ambient pressure (AP), meaning we can introduce reactive gases and determine surface chemical states during the reaction under real conditions. The great thing is that we can conduct our AP-XPS experiments under the same temperatures and pressures we use for our environmental TEM studies, allowing us to bridge structure and chemistry information.
For high-temperature reactions, the CFN low-energy electron microscope (LEEM) has been very helpful. We can look at reactions at up to nearly 2,000 degrees Fahrenheit and introduce reactive gases. Most other systems are not stable enough to work under these conditions. We’ve used the LEEM system at the CFN to study reactions with high-temperature materials, like alloys of nickel and aluminum. Such high-temperature alloys are used in jet and rocket engines.
This movie—captured with the CFN in situ low-energy electron microscope (LEEM)—shows the oxidation of a nickel aluminum alloy, NiAl (100), under very high temperature (1551.2 degrees Fahrenheit) and a partial pressure of oxygen (~5 × 10-10 Torr). An oxide strip advances as it encounters multiple substrate steps during its growth. Research published in Proceedings of the National Academy of Sciences 112, E103 (2015).
How are you currently leveraging these capabilities?
I have several ongoing projects. Two of these projects are related to alloys, which provide a way to modify and control the physical and chemical properties of metals. For example, pure aluminum is quite soft—you can easily bend it. But if you add a second element like nickel to form an aluminum-nickel alloy, the material is much stronger mechanically. And while aluminum has a melting temperature of around 600 degrees Celsius, the nickel-aluminum alloy has a melting temperature of 1,600 degrees Celsius.
My first project, which has received continued support from DOE Basic Energy Sciences over the past 10 years and was just renewed for another three years, is to visualize the early stages of oxidation of metals and alloys. I am using in situ capabilities at the CFN to monitor the oxidation of aluminum and nickel-aluminum alloys under practically relevant temperatures and pressures. Ultimately, the goal is to be able to control these gas-surface reactions for a variety of energy-related applications, ranging from mitigating corrosion damage to increasing fuel efficiency.
My second project, funded by the National Science Foundation (NSF), focuses on a phenomenon typical for alloys: surface segregation. When one alloy element segregates at the surface, the surface composition is different from the bulk composition. So, the properties at the surface and bulk are different. For example, minor local changes to composition can lead to drastic changes in properties such as corrosion resistance and catalytic activity. By applying in situ TEM and XPS at the CFN, I hope to understand how surface segregation happens on a microscopic level.
A third project I’m involved in is part of the Northeast Center for Chemical Energy Storage, a DOE Energy Frontier Research Center. This center is directed by Stanley Whittingham, a Distinguished Professor of Chemistry at Binghamton who was awarded the 2019 Nobel Prize in Chemistry for his development of lithium-ion batteries. I’m using in situ and ex situ tools at the CFN to characterize oxides based on nickel and cobalt, leading cathode materials for lithium-ion batteries. The composition of these materials is quite complicated. Theory predicts their capacity should be much higher than what we are achieving experimentally. So, we need to ask, what is causing the capacity to degrade? How we can improve the structural and chemical stability of the cathode to maximize capacity?
An in situ transmission electron microscopy (TEM) movie showing the conversion of copper (I) oxide (Cu2O) to copper (Cu) at the Cu2O/Cu interface under high temperature (662 degrees Fahrenheit) and a partial pressure of hydrogen (4 × 10-2 Torr). Research published in Nature Communications 8, 307 (2017).
You’ve been a longtime user of the CFN and had previous exposure to a national lab through your postdoc at Argonne. From your experience, what is your perspective on the national labs?
The first encounter I had with a national lab was while I was getting my PhD in materials science. I interacted with some of the staff at Lawrence Livermore National Lab and had the opportunity to visit. After my postdoc at Argonne, where I used synchrotron x-ray diffraction and TEM to study the fundamental mechanisms of oxidation, I thought it would be great to find a job where I could have access to similar capabilities for my research. The position at Binghamton matched well with my research background, and I knew the campus wasn’t far from New York City and Brookhaven Lab.
Beyond offering unique facilities, national labs have staff scientists who are experts in their research fields and on specific instruments. My research and that of my students has tremendously benefitted from this expertise. If you look at my publications, most of them have coauthors from national labs, especially Brookhaven. Staff members have helped us adjust our experimental plans to be more productive and understand our experimental results. When I first became a user at the CFN, I was mostly carrying out experiments on electron microscopes. But through interacting with CFN staff, I learned about XPS, other kinds of microscopy, and computational resources available at the CFN to complement my electron microscopy work. At the CFN, you can look at a sample with several different characterization tools and perform modeling and simulation all in one place staffed by experts ready to help.
Are there any advances in instrumentation or capabilities that would take your field to the next level?
Oxidation has been studied for more than 100 years. We developed some understanding of how oxidation happens from studies based on traditional methods, such as measuring the increase in the total weight of a sample after an oxide is formed. But now we have much more powerful in situ capabilities to study this problem and problems in other fields, like catalysis. I think one of the major directions for the future of my field is for scientists to advance these capabilities even further. For instance, if we can improve the space and time resolution, we’ll be able to look at the reaction at an even smaller scale and faster rate. Right now, we can see oxides as they form at the nanometer scale and monitor the growth process. But with higher resolution, we may be able to see what happens before nucleation begins. I also believe opportunities exist to apply machine learning and artificial intelligence to manage the large datasets generated during in situ studies.
How did you become interested in materials science and oxidation?
Materials are the building blocks for almost everything. Materials have a real impact on our everyday lives, and oxidation can significantly impact how they function. To control oxidation, we must develop a mechanistic understanding at the atomic level. I enjoy applying recent advances in instrumentation to develop new fundamental insights to an old problem.
Interested in becoming a CFN user? Submit a proposal through the CFN Proposal Portal. The next deadline is September 30. If you have questions about using CFN facilities or partnering with CFN scientists, please contact CFN Assistant Director for Strategic Partnerships Priscilla Antunez at (631) 344-6186 or pantunez@bnl.gov.
Brookhaven National Laboratory is supported by the U.S. Department of Energy’s Office of Science. The Office of Science is the single largest supporter of basic research in the physical sciences in the United States and is working to address some of the most pressing challenges of our time. For more information, visit https://energy.gov/science.
Follow @BrookhavenLab on Twitter or find us on Facebook.
2021-19134 | INT/EXT | Newsroom