Project Portfolio
Enabling ultrafast detectors with low-gain avalanche diodes
Low-gain avalanche diodes (LGADs) are a recently developed class of silicon sensors with high time resolution—about 30 ps at room temperature. With fast electronics (~1 GHz), signals can develop in less than 2 ns. To produce fast signals, LGADs are typically fabricated on thin (about 50 mm), high-resistivity silicon wafers and feature a charge multiplication layer for signal amplification.
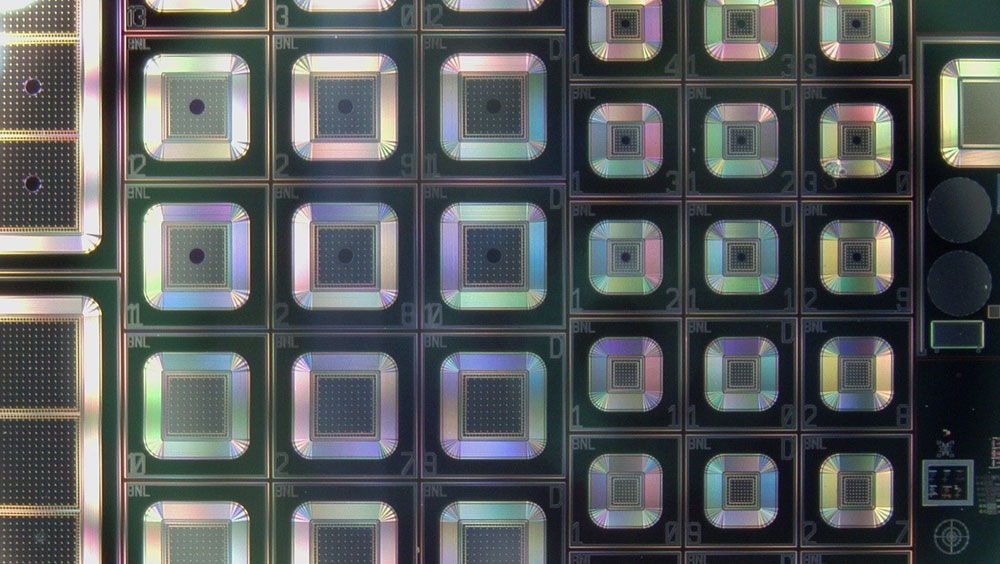
A microscope image of single-pad LGAD devices, with areas of 2 mm × 2 mm (left) and 1 mm × 1 mm (right).
Because of their excellent timing capability, LGADs have attracted the interest of the high-energy physics (HEP) community for particle detection and tracking. For example, future lepton colliders will require a time resolution of a few 100 ps to unambiguously identify hit positions belonging to specific particle tracks and generated by the same particle. Similarly, for hadron colliders such as the proton-proton Future Circular Collider (FCC-hh) and the High-Energy Large Hadron Collider (HE-LHC), timing information of 10 ps or less will be necessary to reject the large background of particles from spurious collisions. Currently, the ATLAS and CMS detectors at the LHC are being upgraded to prepare for the High-Luminosity LHC. A High-Granularity Timing Detector (HGTD) and a Minimum Ionizing Particle Timing Detector (MTP) have been proposed for this upgrade. Both detectors will be made by arrays of 1.3 mm × 1.3 mm LGADs.
Once the LGADs are patterned to square-shaped pads, which are placed into an array, 2-D spatial information can be obtained. However, for a uniform multiplication to occur along the entire pixel surface, the pad dimension must be far larger than that of the substrate thickness. Moreover, at the pixel border of each individual unit (electrode, pixel, strip) and between units are inactive blind regions. Pixel and strip detectors feature a pitch (distance between two pixels) on the order of 100 mm or less. Because the interpixels or interpads and their border regions account for more than 20% of the sensor surface area, if they are built with LGAD technology, these parts of the sensor surface are inactive.
In 2016, the Santa Cruz Institute of Particle Physics developed a novel concept—the capacitively coupled (AC) LGAD—to preserve the fast timing capabilities and ensure a highly segmented detector. Signals are capacitively induced on metal pads placed on a thin insulating layer, which is grown over a large area on a uniform LGAD pad. Leveraging our expertise in standard direct-coupling (DC) LGADs fabrication, we fabricated the first AC-LGADs based on this entirely new concept. Within only a few months, we fine-tuned our existing technology process flow and sensor layout to meet challenging technical requirements. For example, we performed numerical simulations to calculate the different concentrations of dopants (small quantities of other elements added to a material to manipulate its electrical conductivity) required to form junctions in the AC-LGADs with respect to DC-LGADs.
Through lab tests using radioactive sources, we demonstrated the functionality of our fabricated AC-LGADs, which showed pulse amplitudes as high as that of large-area DC-LGADs for the same gain. Pulse amplitude is the main parameter defining the quality of an LGAD, in turn defining other functional characteristics such as timing. In test beams, we verified spatial resolutions comparable to that of same-pitch devices and timing resolutions similar to that of LGADs, as predicted by theoretical studies and numerical simulations. Therefore, our AC-LGADs are a strong candidate for 4-D detectors in applications where space and time information are crucial, such as particle tracking in future HEP experiments. We are continuing to optimize various design parameters to support these applications. We are also proposing that this detector technology be used for the Roman pots—stations of tracking detectors that measure the total cross section of two particle beams in a collider—of the Electron-Ion Collider (EIC) under construction at Brookhaven Lab.
Examples from our project portfolio
-
We fabricated the first capacitively coupled (AC) low-gain avalanche diodes (LGADs) based on a new design concept that preserves fast timing capabilities, making these silicon sensors promising candidates for particle tracking in future high-energy physics experiments.
-
Our co-invention of the silicon drift detector (SDD) has enabled unsurpassed energy resolution and high count rates for a variety of scientific and industrial applications, including particle physics, astrophysics, mining, and soil surveying.
-
We are developing a detector based on thallium bromide (TlBr)—an emerging semiconductor material with excellent radiation-detection properties—to determine the location and content of radioactive sources that emit gamma rays.
-
In support of future astrophysics missions that will probe black holes and other spectacular objects in the universe, we are developing ultrathick cadmium zinc telluride (CZT) detectors with high energy and position resolution.
-
We have been conceptualizing, designing, constructing, and qualifying the sensor array and electronics for Rubin Observatory’s Simonyi Survey Telescope, which will provide an unprecedented view of the universe.
-
We fabricated photocathodes for generating electron bunches to cool ion beams at Brookhaven’s Relativistic Heavy Ion Collider. This electron cooling will maximize collision rates, especially at low energies, enabling scientists to explore how nuclear matter exists in different phases.
The Instrumentation Department is part of Brookhaven Laboratory's Discovery Technologies Directorate.