Project Portfolio
Improving energy resolution with silicon drift detectors
In 1984, the Instrumentation Department and collaborators from the Polytechnic University of Milan revolutionized the detector world with the invention of the silicon drift detector (SDD).
The SDD was revolutionary because of the extremely small-sized anode at its center. Electrons generated by ionizing radiation are driven by an electric field, parallel to the surface of the wafer, toward this low-capacitance anode. The anode dimension is independent of the detector active area and ultimately limited only by the type of interconnection with the readout electronics. The low capacitance and thus low noise allow for unsurpassed energy resolution and high-count rates. Even when reading out at much slower rates, standard silicon diodes cannot rival the low-noise performance of SDDs.
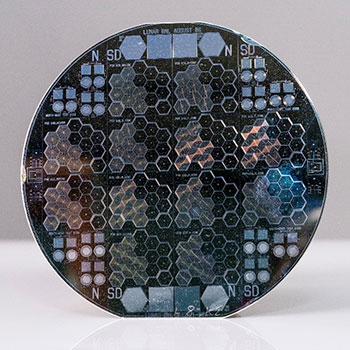
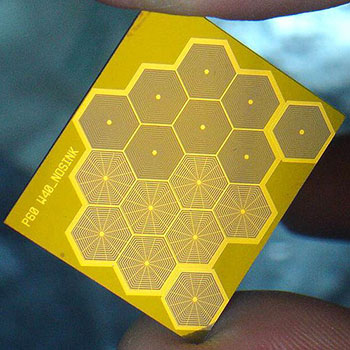
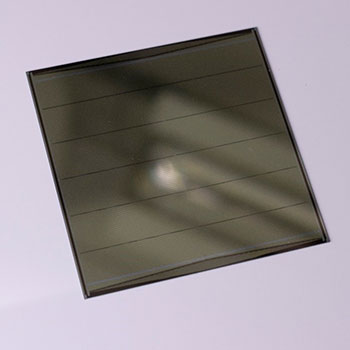
The 4” wafer populated with arrays of SDDs (left) has a uniform thin entrance window on the back. A chip cut from this wafer is shown in the middle. At right is a multi-anode linear SDD for the STAR experiment at RHIC.
However, one drawback of the SDD design was the need for a refined technology process flow to keep a low dark-current level to maintain this low-noise performance. Dark current refers to electrical current thermally generated in the device itself, in the absence of input radiation. Though moderate cooling could lower dark current, such cooling is impractical or unfeasible when the power budget is limited (e.g., in handheld devices) or the dark current is simply too high. Using our in-house fabrication facility, we rapidly put different processes under test to find the optimal process for producing low-current SDDs.
Notably, SDDs were initially used in the particle tracking systems for the STAR experiment at Brookhaven’s Relativistic Heavy Ion Collider (RHIC) and the ALICE experiment at CERN. Using a limited number of readout channels as compared to strip or pixel sensors, the SDDs allowed precise and unambiguous 2-D reconstructions of the hit positions of minimum ionizing particles emerging from the interaction region.
Within a few years, the SDD extended its reach from scientific to industrial applications such as mining, metallurgy, glass and ceramic manufacturing, cement production, and soil surveying.
The SDD has since become a mainstay in x-ray spectroscopy. This technique requires extremely low-noise levels to measure low-energy x-rays from geological, biological, artwork, and other samples of interest. Currently, we are replacing the array of standard PIN diodes in the x-ray microprobe Maia—a high-throughput x-ray fluorescence detector developed at Brookhaven Lab—with an equally large array of small SDDs. This upgrade will allow scientists to reach higher energy resolutions at higher rates, enabling detection of low-energy x-rays and reducing experimental times.
The SDD has also been applied to soft x-ray spectroscopy for astrophysics. To support this application, we developed large-area arrays of SDDs featuring a uniform thin-entrance window on the side of the wafer opposite to the anode. The window must be sufficiently thin to detect soft x-rays absorbed in silicon in a very shallow region. By customizing the design of these arrays for specific astrophysics missions, we have enabled astrophysicists to expand our knowledge of both nearby objects such as the moon and distant, very hot objects created in the early universe.
Examples from our project portfolio
-
We fabricated the first capacitively coupled (AC) low-gain avalanche diodes (LGADs) based on a new design concept that preserves fast timing capabilities, making these silicon sensors promising candidates for particle tracking in future high-energy physics experiments.
-
Our co-invention of the silicon drift detector (SDD) has enabled unsurpassed energy resolution and high count rates for a variety of scientific and industrial applications, including particle physics, astrophysics, mining, and soil surveying.
-
We are developing a detector based on thallium bromide (TlBr)—an emerging semiconductor material with excellent radiation-detection properties—to determine the location and content of radioactive sources that emit gamma rays.
-
In support of future astrophysics missions that will probe black holes and other spectacular objects in the universe, we are developing ultrathick cadmium zinc telluride (CZT) detectors with high energy and position resolution.
-
We have been conceptualizing, designing, constructing, and qualifying the sensor array and electronics for Rubin Observatory’s Simonyi Survey Telescope, which will provide an unprecedented view of the universe.
-
We fabricated photocathodes for generating electron bunches to cool ion beams at Brookhaven’s Relativistic Heavy Ion Collider. This electron cooling will maximize collision rates, especially at low energies, enabling scientists to explore how nuclear matter exists in different phases.
The Instrumentation Department is part of Brookhaven Laboratory's Advanced Technology Research Office.