Project Portfolio
Detecting gamma rays with TlBr
Thallium bromide (TlBr) is an emerging semiconductor material for gamma-ray detectors. Detectors based on TlBr have higher spectral resolution, higher detection efficiency, and significantly lower fabrication costs compared to those of any other room-temperature semiconductor material. Unlike high-purity germanium detectors, TlBr detectors do not require expensive cooling to cryogenic temperatures for operation. As a detecting medium, TlBr has inherently low leakage current and low statistical fluctuations of output signals because it operates by collecting charges rather than light, as is the case for scintillator materials. With these properties, TlBr has a much better energy resolution than that of scintillator materials such as sodium iodide and lanthanum bromide, which are commonly used in handheld gamma-ray detectors.
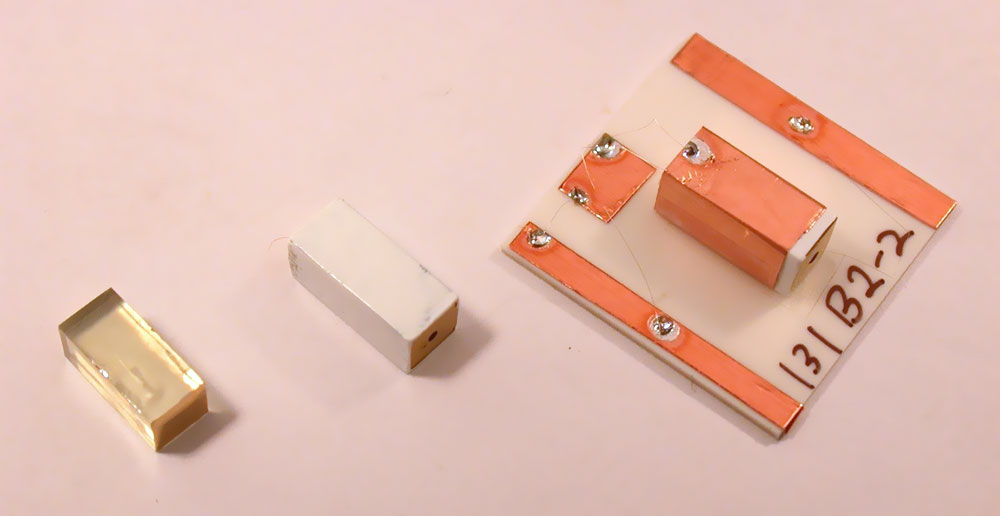
At left, a 5 × 5 × 12 mm3 TlBr crystal precut for integration into a detector. Courtesy of Radiation Monitoring Devices.
A broad application area exists for TlBr detectors—from gamma-ray astronomy to medical imaging and nuclear security. However, large-volume TlBr crystals suffer from charge carrier losses due to crystal defects. Improving the charge-carrier efficiency is important because the more charges that can be collected, the larger the signals that can be obtained from the detector. Until recently, TlBr detectors with thicknesses greater than 2–3 mm were not practically viable due to severe charge losses. Improvements in crystal growth techniques made by companies such as Radiation Monitoring Devices (RMD) are now opening opportunities for using thick TlBr crystals for the detection of gamma rays in practical applications.
In response to a U.S. Department of Homeland Security (DHS) need for advanced radiation detectors for nuclear radiation monitoring, we are collaborating with RMD and Bubble Technology Industries (BTI) to develop a high-performance TlBr radionuclide identification device (RIID). An RIID is an instrument designed to locate radioactive sources and determine the identity of the radioactive materials contained in these sources by measuring the energy of the emitted gamma rays. Personnel use RIIDs to interdict movement of radioactive materials and provide situational awareness during radiological emergencies.
Leveraging our expertise in radiation detectors and readout electronics, we proposed a novel detector design to overcome the poor charge collection in TlBr. Our gamma-ray detector is composed of a 3 ´ 3 array of bar-shaped TlBr crystals, which are configurated as position-sensitive virtual Frisch-grid (VFG) detectors and coupled with state-of-the-art low-noise front-end electronics based on application-specific integrated circuits (ASICs) being developed by our in-house ASIC Development Group. Position-sensitive VFG refers to a particular detector design in which metal contacts are arranged in a particular geometry. This geometry ensures that signals generated by gamma rays can be read out and used to measure the energies and locations of their interactions inside the detector.
Our proposed TlBr detectors employ already proven technology that has been applied to cadmium zinc telluride (CdZnTe, or CZT), another attractive material for gamma-ray detectors. This technology allows for the amplitudes of the signals generated inside the TlBr crystals to be corrected. Due to crystal defects, nonuniform signal response is common in TlBr and many other compound semiconductor materials. The correction enhances the sensitivity of the detector to radionuclides; improves the energy resolution of the detector, or its ability to distinguish between different radionuclides; and reduces material production costs.
We also developed the corresponding readout electronics and digital signal processing system. Our complex signal processing algorithm will enhance position and energy resolutions, which have a transformational impact on the performance of handheld radiation detectors. Working with RMD and BTI, we will help design the TlBr detector and electronics for the RIID in a ruggedized, weatherproof package of optimal size, weight, and power to meet DHS field operation requirements.
Examples from our project portfolio
-
We fabricated the first capacitively coupled (AC) low-gain avalanche diodes (LGADs) based on a new design concept that preserves fast timing capabilities, making these silicon sensors promising candidates for particle tracking in future high-energy physics experiments.
-
Our co-invention of the silicon drift detector (SDD) has enabled unsurpassed energy resolution and high count rates for a variety of scientific and industrial applications, including particle physics, astrophysics, mining, and soil surveying.
-
We are developing a detector based on thallium bromide (TlBr)—an emerging semiconductor material with excellent radiation-detection properties—to determine the location and content of radioactive sources that emit gamma rays.
-
In support of future astrophysics missions that will probe black holes and other spectacular objects in the universe, we are developing ultrathick cadmium zinc telluride (CZT) detectors with high energy and position resolution.
-
We have been conceptualizing, designing, constructing, and qualifying the sensor array and electronics for Rubin Observatory’s Simonyi Survey Telescope, which will provide an unprecedented view of the universe.
-
We fabricated photocathodes for generating electron bunches to cool ion beams at Brookhaven’s Relativistic Heavy Ion Collider. This electron cooling will maximize collision rates, especially at low energies, enabling scientists to explore how nuclear matter exists in different phases.
The Instrumentation Department is part of Brookhaven Laboratory's Discovery Technologies Directorate.