Relativistic Heavy Ion Collider Begins 18th Year of Experiments
First smashups with 'isobar' ions and low-energy gold-gold collisions will test earlier hints of exciting discoveries as accelerator physicists tune up technologies to enable future science
March 21, 2018
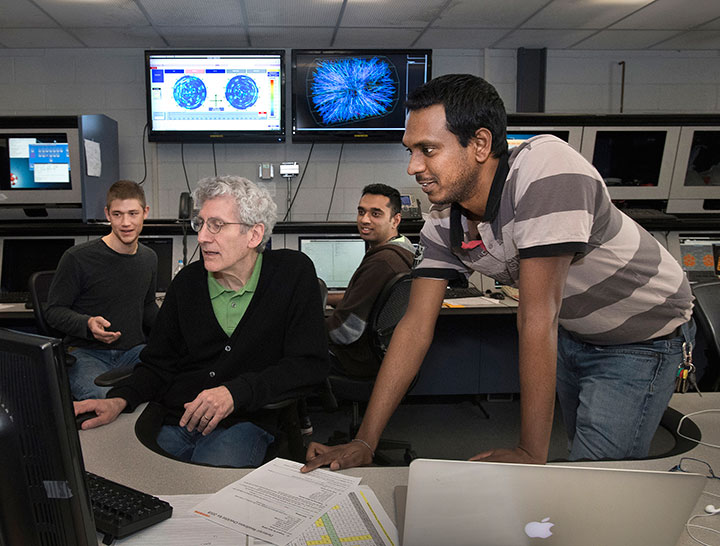
Members of the STAR collaboration in the STAR control room on shift during this year's physics run, with STAR's event plane detector graphic user interface and particle tracks in the time projection chamber on display behind them: front, l to r: shift leader Carl Gagliardi of Texas A&M University with shift leader trainee Prashanth Shanmuganathan, a postdoctoral associate at Lehigh University; rear, l to r: Joseph Adams a graduate student at Ohio State University and Raghav Kunnawalkam Elayavalli a postdoctoral fellow at Wayne State University. Adams and Shanmuganathan worked on construction of the event plane detector and serve as detector experts; Kunnawalkam Elayavalli has been controlling the event plane detector during STAR data taking as a detector operator trainee.
UPTON, NY— The first smashups of two new types of particles at the Relativistic Heavy Ion Collider (RHIC)—a U.S. Department of Energy (DOE) Office of Science user facility for nuclear physics research at Brookhaven National Laboratory—will offer fresh insight into the effects of magnetism on the fireball of matter created in these collisions. Accomplishing this main goal of the 15-week run of RHIC’s 18th year will draw on more than a decade of accumulated expertise, enhancements to collider and detector components, and a collaborative effort with partners across the DOE complex and around the world.
In some ways this run is the culmination of two decades of facility development.”
— Wolfram Fischer, Collider-Accelerator Department
Physicists will also perform two different kinds of collisions with gold ions at low energies, including collisions of gold ions with a stationary target. These collisions will help scientists better understand the exotic matter created in RHIC’s highest energy collisions, including the strength of its magnetic field and how it evolves from a hot soup of matter’s fundamental building blocks (quarks and gluons) to the ordinary protons and neutrons that make up the bulk of visible matter in the universe today.
As an added bonus—or rather, a testament to the efficiency of RHIC accelerator staff—the collider-accelerator team will also be implementing and fine-tuning several technologies important for future nuclear physics research.
“In some ways this run is the culmination of two decades of facility development,” said Wolfram Fischer, Associate Chair for Accelerators in Brookhaven Lab’s Collider-Accelerator (C-AD) Department. “We will make use of many tools we have developed over many years, which we now need all at the same time. All this expertise in C-AD and support from DOE and other labs came together to make this possible.”
Helen Caines, a physicist at Yale University who serves as co-spokesperson for RHIC’s STAR experiment, agreed and expressed her appreciation for RHIC’s unique versatility and ability to pack in so much in such a short time. “It’s going to be a busy 15 weeks!” she said.
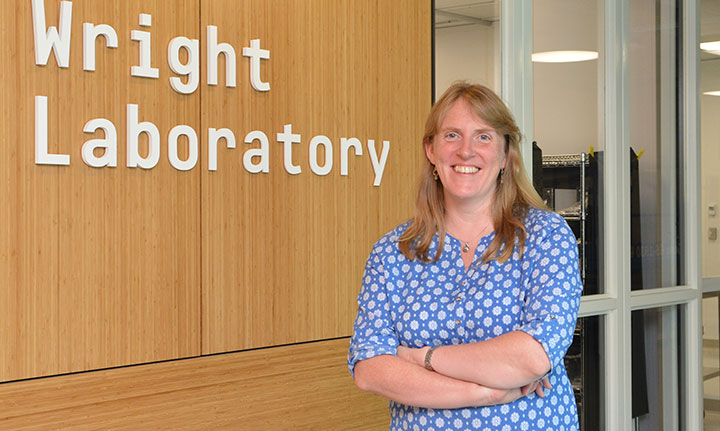
STAR experiment co-spokesperson Helen Caines is a member of Wright Lab, a Yale University center for instrumentation development, research, and teaching programs focused on nuclear, particle, and astrophysics. Credit: Yale University
Studying magnetic effects
RHIC collides ions (for example, the nuclei of heavy atoms such as gold that have been stripped of their electrons) to “melt” their protons and neutrons and set free those particles’ internal building blocks, known as quarks and gluons. Creating this “quark-gluon plasma” mimics the conditions of the very early universe and gives scientists a way to explore the force that governs how these fundamental particles interact. The nuclear physicists conduct these studies by tracking the particles emerging from the collisions.
One intriguing finding from an earlier run at RHIC was an observation of differences in how negatively and positively charged particles flow out from the fireball created when two gold ions collide. Scientists suspect that this charge separation is triggered in part by something called the “chiral magnetic effect”—an interaction between the powerful magnetic field generated when the positively charged ions collide slightly off center (producing a swirling mass of charged matter) and each individual particle’s “chirality.” Chirality is a particle’s right- or left-handedness, which depends on whether it is spinning clockwise or counterclockwise relative to its direction of motion. According to this understanding, the charge separation should get stronger as the strength of the magnetic field increases—which is exactly what STAR scientists are testing in Run 18.
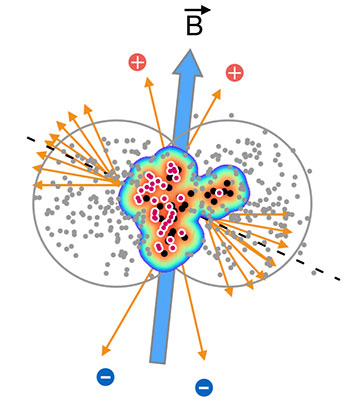
In gold-gold collisions, RHIC physicists observed a separation of charges that they believe was triggered in part by the powerful magnetic field (blue arrow, B) created by the swirl of positively charged particles in off-center collisions
“Instead of gold, we are using collisions with two different ‘isobars’—isotopes of atoms that have the same mass but different numbers of protons, and therefore different levels of positive charge,” said Caines. Collisions of two ruthenium ions (mass number 96 with 44 protons) will create a magnetic field that’s 10 percent stronger than collisions of two zirconium ions (mass number 96 with only 40 protons), she said.
“We are keeping everything else the same—the size of nucleus, the energy, and the total number of particles participating in the collision. We’ll even be switching from one ion species to the other on close to a day-by-day basis to eliminate any variation running the two types of collisions weeks apart might cause. Since the only thing we are varying is the magnetic field, this should be a definitive test of the chiral magnetic effect.”
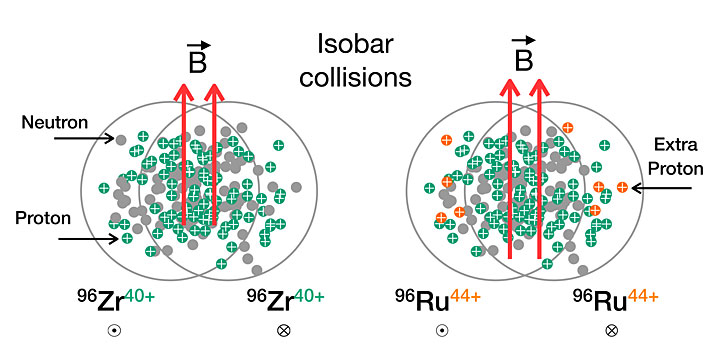
In the isobar collisions, the physicists will vary the strength of the magnetic field by colliding different kinds of ions with the same overall number of nucleons but different numbers of protons, and therefore positive charge. The stronger field produced in the ruthenium collisions (right) should result in a stronger charge-separation effect than the weaker field created in zirconium collisions (left).
A positive result would prove that the collisions are creating a very strong magnetic field—“the strongest ever observed,” Caines said. “It would also be definitive proof that the collisions are creating a medium made up of free quarks and gluons, a quark-gluon plasma, with an imbalance of left- and right-handed particles driven by quantum fluctuations.”
Obtaining and prepping the isotopes
Though the amount of matter needed to collide individual ions is extremely small (RHIC will use much less than a gram of gold in all its years of operation!), obtaining certain rare isotopes can be challenging. Zirconium-96 (the form needed for these experiments) makes up less than three percent of the naturally occurring supply of this element, while ruthenium-96 makes up less than six percent.
“If you just used natural material for the ion sources that feed RHIC, the beam intensity would be way too low to collect the data needed,” said Fischer. “You can buy enriched samples of zirconium but there is no commercial source of enriched ruthenium.”
Fortunately, there is a new facility for such isotope enrichment at DOE’s Oak Ridge National Laboratory (ORNL), the Enriched Stable Isotope Prototype Plant (ESIPP), which heated up the natural material and electromagnetically separated out the different masses. ESIPP is part of the DOE Isotope Program and started operations in FY 2018, re-establishing a general domestic capability to enrich stable isotopes.
“With the help of the DOE Isotope Program in the Office of Science, ORNL put us at the top of their priority list to provide one-half gram of this material—a little vial with a bit of ‘dust’ in the bottom—in time for the run,” Fischer said.
The ruthenium ions start their path of acceleration in Brookhaven’s Tandem Van De Graaff accelerator. So as not to waste any of the precious ion supply, the Tandem team, led by Peter Thieberger, first ran tests with higher-abundance forms of ruthenium, making sure they’d have the beam intensity needed. For the actual experiments, they dilute the ruthenium sample with aluminum to spread out the supply. Once accelerated, the ions get bunched and those bunches get combined into more and more tightly pack bunches as they circulate through the Booster ring and the Alternating Gradient Synchrotron (AGS), gaining energy at each step before being injected into RHIC’s two counter-circulating 2.4-mile-circumference rings for collisions at 200 billion electron volts (GeV).
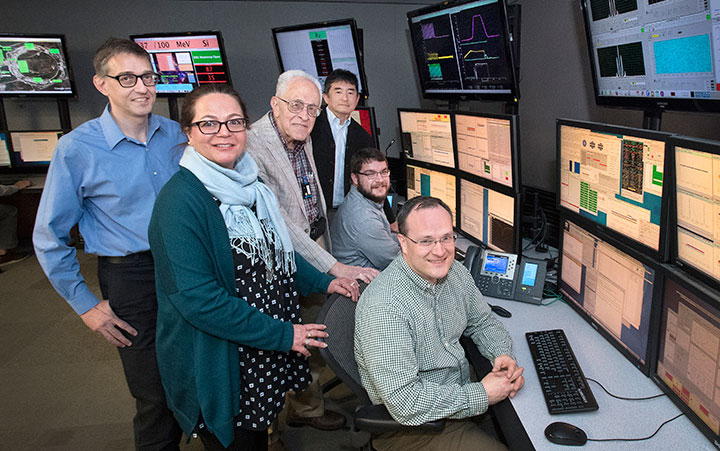
Wolfram Fischer, left, in the Collider-Accelerator Department Main Control Room with leaders of the various programs that were essential to the isobar run at RHIC this year. Pictured standing left to right are: Cathy Cutler, Peter Thieberger, Masahiro Okamura; seated back to front: Kevin Mernick and Greg Marr.
To get the zirconium ions for collisions on the alternating days, the Brookhaven team, led by Masahiro Okamura, sought help from Hiromitsu Haba and colleagues at Japan’s RIKEN laboratory who’d had experience with zirconium targets. “They generously shared everything they know about transforming zirconium into oxide targets we could use to extract the ions,” Fischer said.
Scientists zap these zirconium oxide targets with a laser at Brookhaven’s Laser Ion Source to create a plasma containing positively charged zirconium ions. Those ions then enter the Electron Beam Ion Source (EBIS) to be transformed into a beam. From EBIS, the zirconium beam follows a path similar to that of ruthenium, with the ions merging into tighter and tighter bunches and gaining energy in the Booster and AGS before being injected into RHIC. Yet another team—Brookhaven’s own chemists from the Medical Isotope Research and Production Program, led by Cathy Cutler—recovers leftover target material and reprocess it to make new targets so that no valuable isotope material is left unused.
Having the two types of ions enter RHIC from different sources makes it easier to switch from ruthenium to zirconium day by day. “These are two somewhat exotic species of ions, so we wanted two independent sources that can be optimized and run independently,” Fischer said. “If you run both out of one source, it’s harder to get the best performance out of both of them.”
Once either set of ions enters the collider, additional enhancements made at RHIC over the years help maximize the number of data-producing collisions. Most significantly, a technique called “stochastic cooling,” implemented during this run by Kevin Mernick, detects when particles within the beams spread out (heat up), and sends corrective signals to devices ahead of the speeding ions to nudge them back into tight packs.
“Without stochastic cooling it would be very hard if not impossible to reach the experimental goals because we would lose a lot of ions,” Fischer said. “And we couldn’t do this without all the different parts in DOE and at Brookhaven. We needed all our source knowledge in EBIS and at the Tandem, and we needed collaborators from RIKEN, ORNL, and our chemists in the Isotope Program at Brookhaven as well. It’s been an amazing collaborative effort.”
“Switching from one species to another every day has never been done before in a collider,” Fischer said. “Greg Marr, the RHIC Run Coordinator this year, needs to draw on all tools available to make these transitions as quickly and seamlessly as possible.”
More to learn from gold-gold
Following the isobar run, STAR physicists will also study two kinds of gold-gold collisions. First, in collisions of gold beams at 27 GeV, they will look for differential effects in how particles called lambdas and oppositely charged antilambda particles emerge. Tracking lambdas recently led to the discovery that RHIC’s quark-gluon plasma is the fastest spinning fluid ever encountered. Measuring the difference in how lambdas and their antiparticle counterparts behave would give STAR scientists a precise way to measure the strength of the magnetic field that causes this “vorticity.”
“This will help us improve our calculations of the chiral magnetic effect because we would have an actual measurement of the magnetic contribution. Until now, those values have been based purely on theoretical calculations,” Caines said.
In the final phase of the run, accelerator physicists will configure RHIC to run as a fixed-target experiment. Instead of crashing two beams together in head-on collisions, they will slam one beam of gold ions into a gold foil placed within the STAR detector. The center of mass collision energy, 3.2 GeV, will be lower than in any previous RHIC run. These collisions will test to see if a signal the scientists saw at higher energies—large fluctuations in the production of protons— turns off. The disappearance of this signal could indicate that the fluctuations observed at higher energies were associated with a so-called “critical point” in the transition of free quarks and gluons to ordinary matter. The search for this point—a particular set of temperature and pressure conditions where the type of phase transformation changes—has been another major research goal at RHIC.
These lowest energy collisions will also form the start of the next “beam energy scan,” a series of collisions across a wide range of energies beginning in earnest next year, Caines said. That work will build on results from earlier efforts to map the various phases of quark-gluon matter.
Tuning up detector and accelerator technologies
Some newly upgraded components of the STAR detector will be essential to these and future studies of nuclear matter at RHIC, so STAR physicists will be closely monitoring their performance during this run. These include:
- An inner component of the barrel-shaped Time Projection Chamber (the iTPC), developed with significant support from DOE and China’s National Natural Science Foundation and Ministry of Science and Technology.
- An “endcap time of flight” (eTOF) detector developed by STAR physicists and a collaboration of scientists working on the Compressed Baryonic Matter experiment, which will be located at the future Facility for Antiproton and Ion Research in Darmstadt, Germany.
- A new “event plane detector” developed by U.S. and Chinese collaborators in a project supported by the DOE, the U.S. National Science Foundation, and the Chinese Ministry of Science and Technology.
The first two of these components work together to track and identify particles emerging from collisions closer to the beamline than ever before, enabling physicists to more precisely study directional preferences of particles. The event plane detector will track the orientation of the overlap region created by colliding particles—and therefore the orientation of the magnetic field.
“The combination of these new components will enhance our ability to track and identify particles and study how the patterns of particles produced are influenced by collision conditions,” Caines said.
On the accelerator front, Fischer notes two major efforts taking place in parallel with the Run 18 physics studies.
One project is commissioning a newly installed electron accelerator for low energy electron cooling, an effort led by Alexei Fedotov. This major new piece of equipment uses a green-laser-triggered photocathode electron gun to produce a cool beam of electrons. The electrons get injected into a short section of each RHIC ring to mix with the ion beams and extract heat, which reduces spreading of the ions at low energies to maximize collision rates.
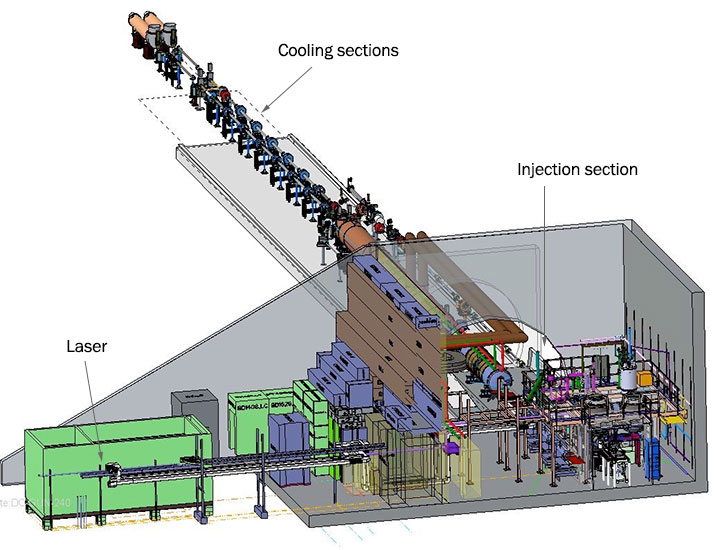
Schematic of low-energy electron cooling components.
The commissioning will include fine tuning the photocathode gun and the radiofrequency (RF) cavities that accelerate the electron beam after it leaves the gun to get it up to speed of RHIC’s gold beams. The physicists will also commission RF correctors that give extra kicks to lagging particles and slow down those that are too speedy to keep all the electrons closely spaced.
“We have to make sure the electron beam has all the necessary properties—energy, size, momentum spread, and current—to cool the ion beam,” Fischer said. “If everything goes right, then we can use this system to start cooling the gold beam next year.”
Physicists will also test another system for electron cooling at higher energies, which was developed in an effort led by Vladimir Litvinenko. In this system, called coherent electron cooling, electron beams are used as sensors for picking up irregularities in the ion beam. “The electron beam gets ‘imprinted’ by regions of low or high ion density,” Fischer said. Once amplified, this signal in the electron beam can be fed back to the ion beam “out of phase” to smooth out the irregularities.
Though this type of cooling is not essential to the research program at RHIC, it would be essential for cooling beams in a high-energy Electron-Ion Collider (EIC), a possible future research facility that nuclear physicists hope to build. Testing the concept at RHIC helps lay the foundation for how it would work at an EIC, Fischer said.
If the experience at RHIC is any guide, all the testing should pay off with future physics discoveries.
Research at RHIC is funded primarily by the DOE Office of Science, and also by these agencies and organizations.
Brookhaven National Laboratory is supported by the Office of Science of the U.S. Department of Energy. The Office of Science is the single largest supporter of basic research in the physical sciences in the United States, and is working to address some of the most pressing challenges of our time. For more information, please visit science.energy.gov.
Follow @BrookhavenLab on Twitter or find us on Facebook.
2018-12738 | INT/EXT | Newsroom