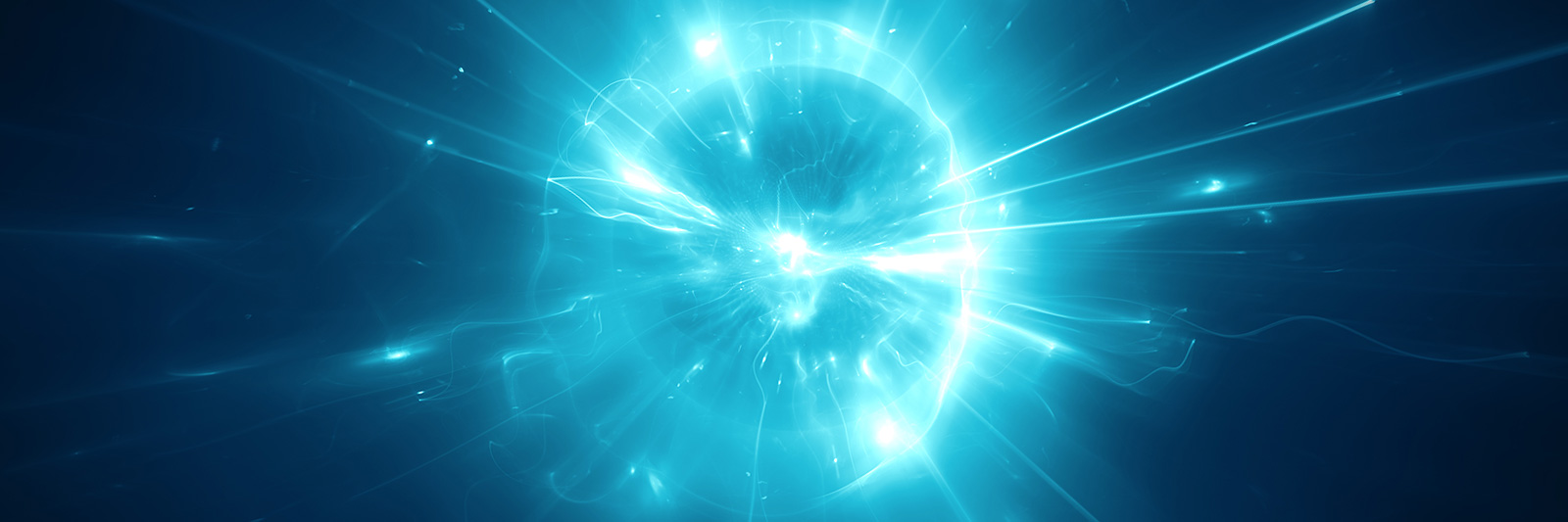
Brookhaven Neutrino Research
Neutrinos, ghostlike particles that flooded the universe just moments after the Big Bang, are born in the hearts of stars and other nuclear reactions. Untouched by electromagnetism and nearly as fast as light, neutrinos pass practically unhindered through everything from planets to people, only rarely responding to the weak nuclear force and the even weaker gravity. In fact, at any given moment, tens of billions of neutrinos are passing through every square centimeter of the Earth’s surface, undetected. This ability to sail unhindered and unnoticed through almost anything earned neutrinos the nickname “ghost” particles. But despite their imperceptibility, neutrinos could be the key to understanding how our universe evolved just after the Big Bang and why the world is made of matter.
The Goldhaber Helicity Experiment
Brookhaven Lab’s first major contribution to neutrino research occurred in 1957, when Maurice Goldhaber performed an experiment that revealed neutrinos to be "left-handed." That is, a property of neutrinos known as "spin" is always oriented counterclockwise to the direction of their linear momentum.
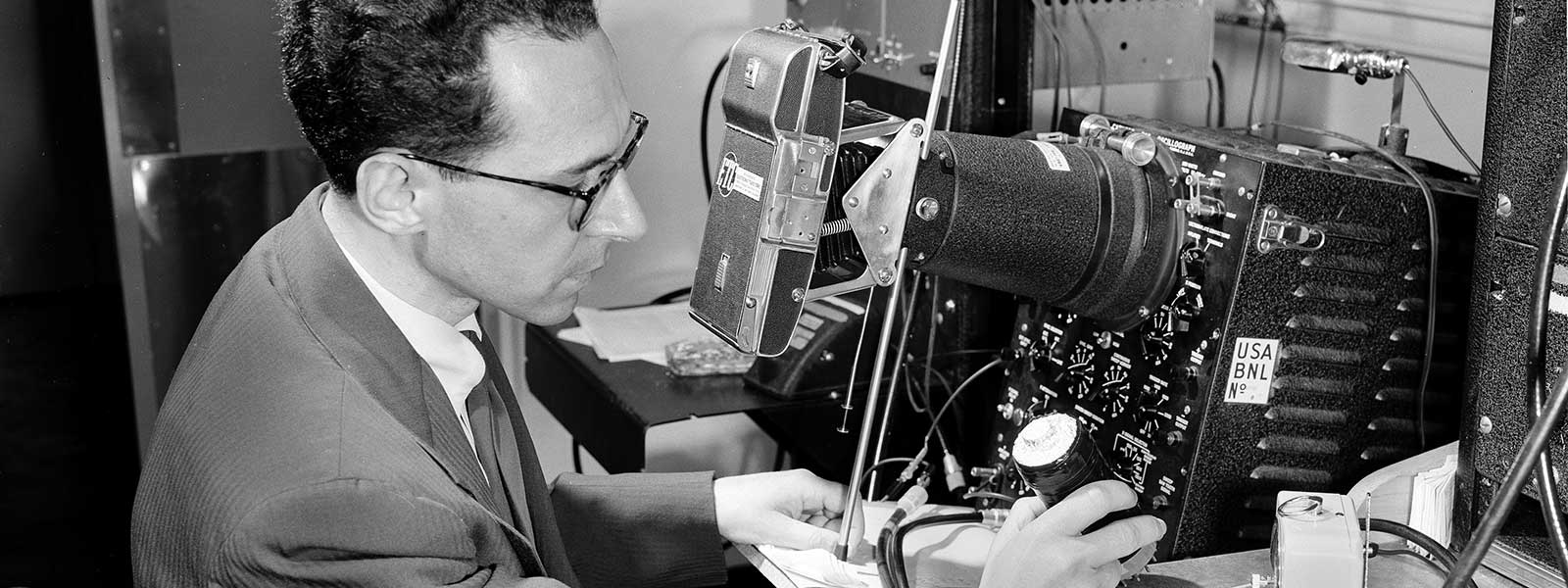
Maurice Goldhaber proved that the spin of neutrinos is always oriented counterclockwise to the direction of their linear momentum. He also contributed to measurement of the mass of the neutron, the discovery of the nuclear photo-effect, the role of spin in nuclear reactions, and a wide variety of additional physics research. He served as Director of Brookhaven National Lab from 1961 to 1973.
Discovery of the Muon Neutrino
In 1962, a new type of neutrino, the muon neutrino, was discovered by scientists using the Alternating Gradient Synchrotron at Brookhaven. Leon Lederman, Mel Schwartz, and Jack Steinberger took home the 1988 Nobel Prize in Physics for this work, which established that there was more than one flavor of neutrino.
The Solar Neutrino Problem
In the late 1960s, Brookhaven chemist Ray Davis discovered the solar neutrino problem. At the Homestake Mine in South Dakota, deep underground in order to shield the detector from cosmic rays, Davis was the first person able to directly detect the electron neutrinos being produced by the sun. But he only observed about one-third of the expected amount — this deficit would eventually become known as the solar neutrino problem (and the “missing” neutrinos would later turn out to be those that had changed to forms undetectable by Davis’ experiment while en route to Earth). Davis was awarded the 2002 Nobel Prize in Physics for his detection of solar neutrinos.
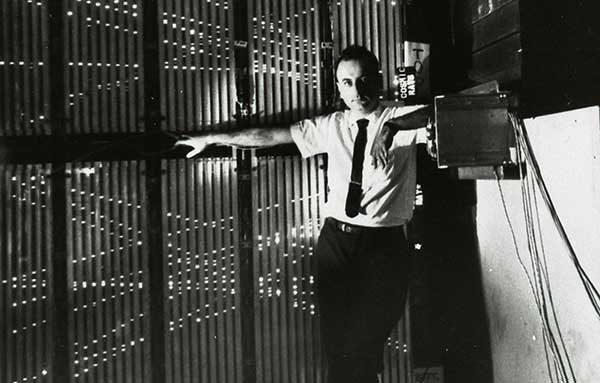
Melvin Schwartz standing next to a neon-filled detector called a "spark chamber" used to produce particle trails that could be photographed to prove the existence of muon neutrinos.
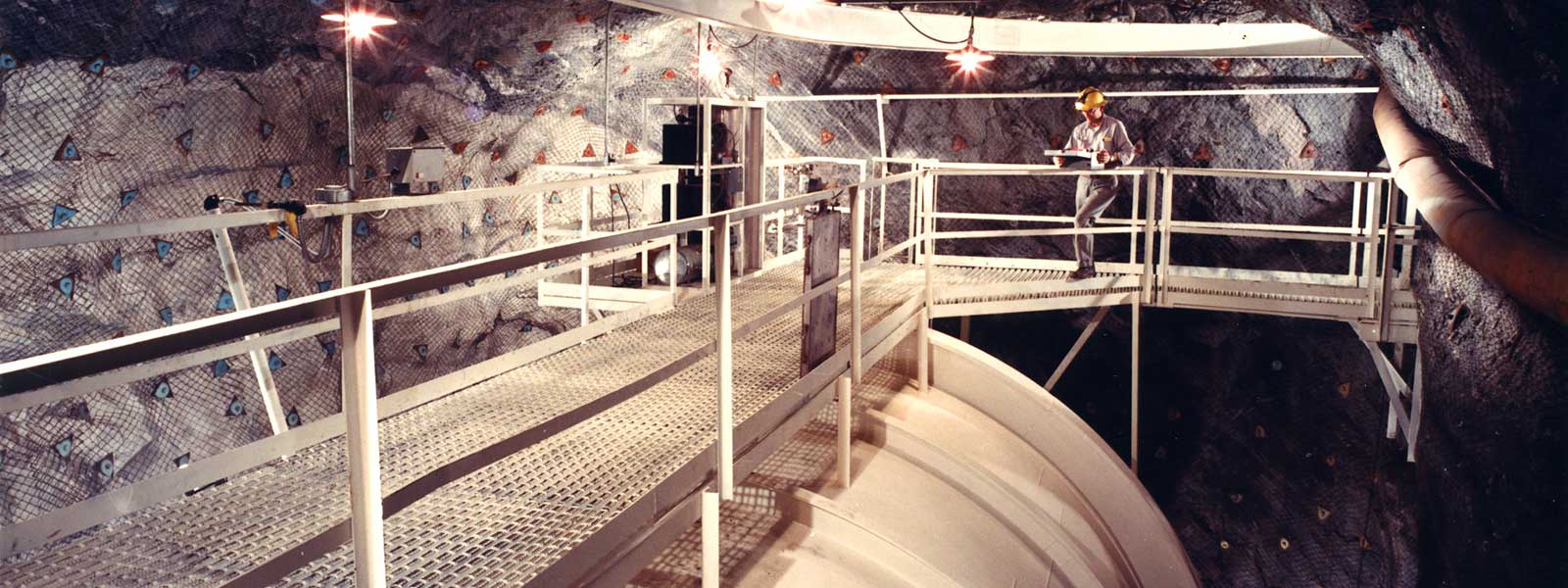
BNL researcher Raymond Davis, Jr. set up a detector in a South Dakota mine to measure neutrinos from the sun as a way to examine the sun's energy generation.
The Irvine-Michigan-Brookhaven (IMB) detector
Brookhaven Lab physicist Maurice Goldhaber was a founding member of a pioneering experiment built in the Morton salt mine in Ohio in the early 1980s that became famous for observing neutrinos from Supernova 1987A (along with the Kamioka detector in Japan). Originally designed to study proton decays, the experiment was a six-story cube lined with black plastic and a network of phototubes and filled with 2.5 million gallons of ultra-pure water. The neutrinos showed up by way of their interactions with protons in the water.
Gallium Experiment (GALLEX) and Sudbury Neutrino Observatory (SNO)
From the 1990s through the mid-2000s, Brookhaven chemists played important roles in the Gallium Experiment (GALLEX) and Sudbury Neutrino Observatory (SNO) in Italy and Canada, respectively. Brookhaven chemist Richard Hahn and his group were integral to the SNO experiment, which proved that neutrinos do oscillate between three forms — electron, muon, and tau. Arthur B. McDonald of Canada’s Queen's University and SNOLAB shared the 2015 Nobel Prize in Physics with Takaaki Kajita, leader of the Super-Kamiokande experiment in Japan, for their work demonstrating that neutrinos change identities, or oscillate.
Super-Kamiokande (Super-K)
The Super-Kamiokande experiment in Japan, in which Brookhaven was represented by physicist Maurice Goldhaber, confirmed that neutrinos do indeed oscillate and have mass. Ray Davis’s “missing” neutrinos problem was solved: he had observed only the fraction of electron neutrinos from the sun that reached Earth without changing into muon or tau neutrinos. Davis's work was acknowledged with the 2002 Nobel Prize in Physics, shared with Masatoshi Koshiba of Japan and Riccardo Giacconi of the U.S. Super-Kamiokande physicist Takaaki Kajita shared the 2015 Nobel Prize in Physics with Arthur B. McDonald of Canada’s Queen's University and SNOLAB for their discovery of neutrino oscillations.
Main Injector Neutrino Oscillation Search (MINOS)
Brookhaven physicists made leading contributions to the Main Injector Neutrino Oscillation Search (MINOS) experiment based at Fermi National Accelerator Laboratory (Fermilab) in Illinois. MINOS began taking data in 2005 and has since provided measurements of mixing angles and oscillation frequency that describe how muon and tau neutrino types oscillate between one form and another.
GALLEX and SNO collaborator Richard Hahn.
Inside the Super-Kamiokande neutrino detector during work on the detectors. (Credit: Kamioka Observatory)
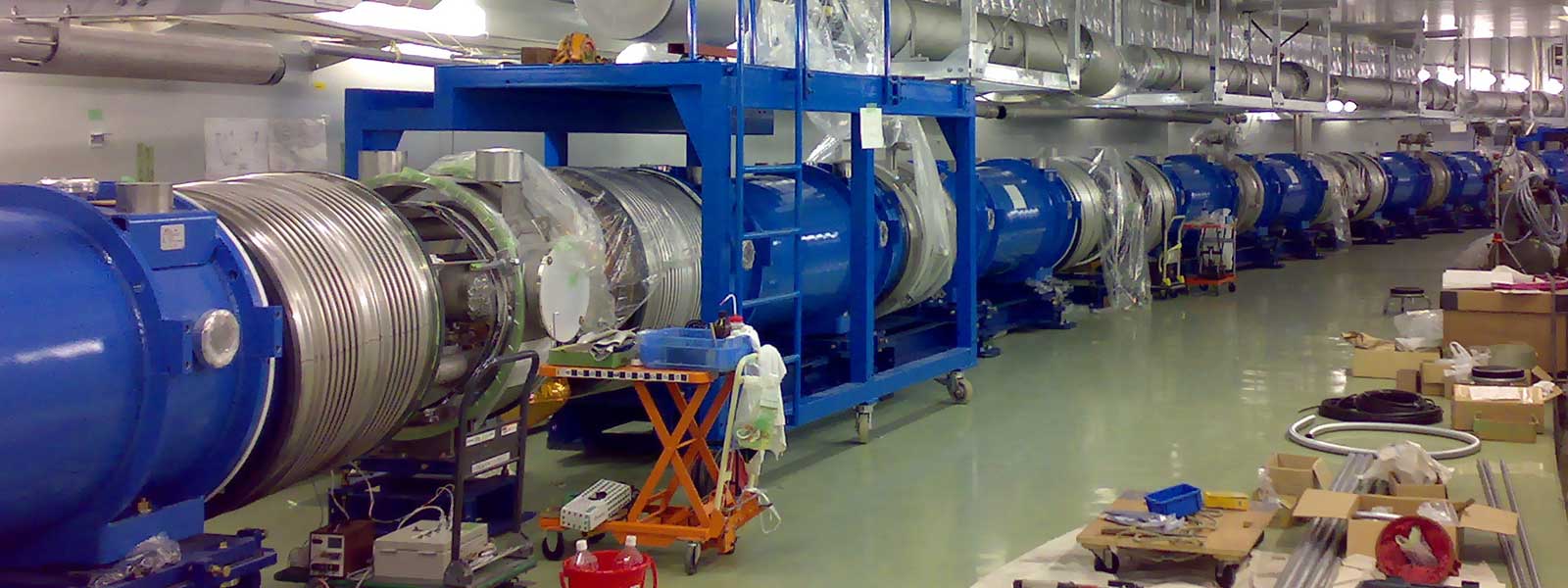
Beamline of the T2K experiment under construction. Brookhaven engineers built five dipole “corrector” magnets, a key part of the experiment. These superconducting magnets are sandwiched between 28 “bending” magnets that drive an intense beam of protons into a stationary target. This high-speed impact creates a shower of particles, some of which decay to neutrinos. (Credit: J-PARC)
Tokai to Kamiokande (T2K)
T2K is another neutrino oscillation experiment that directs an intense beam of muon neutrinos generated at the J-PARC nuclear physics site on the east coast of Japan across the country to the Super-Kamiokande neutrino detector in the mountains of western Japan. Brookhaven scientists and engineers used direct wind machines to make superconducting corrector magnets for the J-PARC beamline that delivers protons to the target for making neutrinos for T2K.
Daya Bay
Brookhaven scientists were integral to the Daya Bay Neutrino Project. This experiment used antineutrinos generated in nuclear reactors in Southern China to measure the final unknown mixing angle that describes how neutrinos oscillate. Daya Bay collaborators were awarded the 2016 Breakthrough Prize in Fundamental Physics for their contributions to this experiment. Brookhaven scientists played multiple roles in the Daya Bay experiment, ranging from management to data analysis. In addition to coordinating detector engineering and design efforts and developing software and analysis techniques, Brookhaven chemists perfected the "recipe" for the gadolinium-doped liquid scintillator used to detect reactor antineutrinos. The experiment collected data from 2011 to 2020. Besides the precision measurement of the neutrino oscillation parameters, Daya Bay also made important measurements of the reactor antineutrino spectrum, fuel evolution, and set limits on the possible existence of so-called sterile neutrinos.
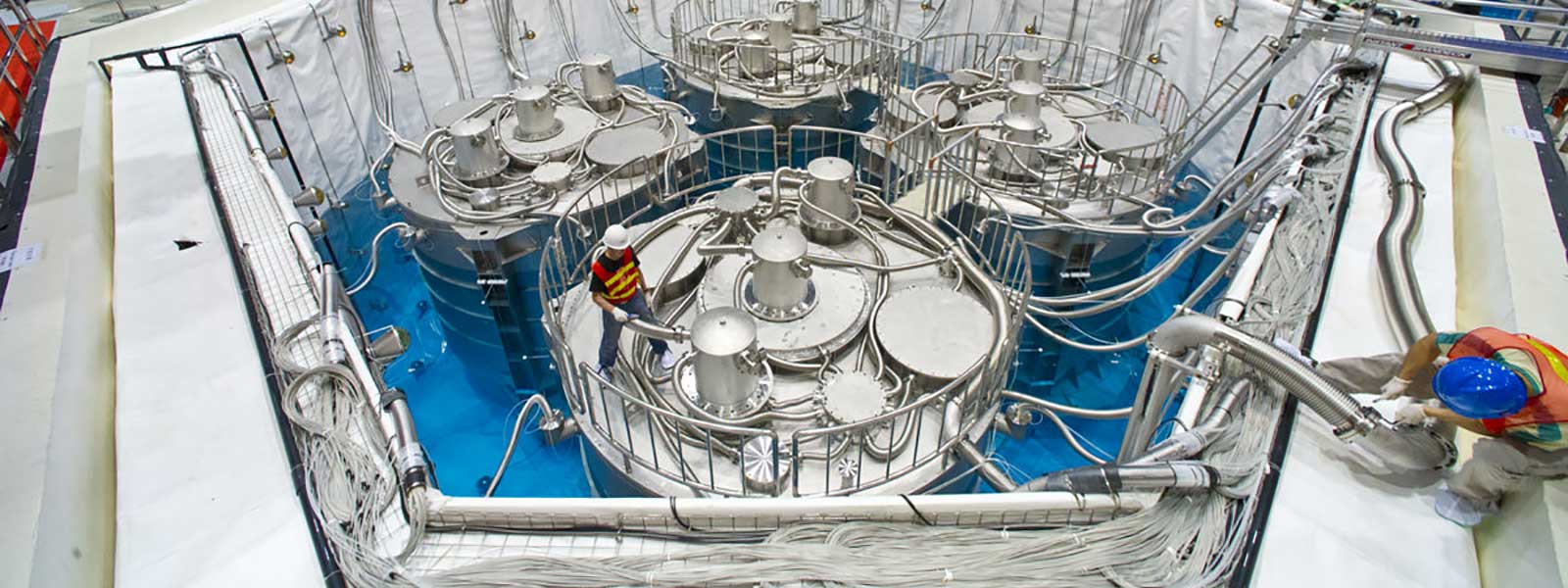
Antineutrino detectors are submersed in liquid at the Daya Bay experiment, as seen during the final phase of construction in August 2012. (Credit: Roy Kaltschmidt/Berkeley Lab)
Micro Booster Neutrino Experiment (MicroBooNE)
Many Brookhaven physicists played leading roles in the design and construction of the MicroBooNE cryogenic neutrino detector, located at Fermilab. MicroBooNE was a state-of-the-art massive liquid argon Time-Projection-Chamber (LArTPC) detector for neutrino physics. The design of LArTPC particle detectors was pioneered by Veljko Radeka and other Brookhaven Lab physicists and engineers. MicroBooNE’s LArTPC included custom charge-readout electronics designed and built by the Brookhaven team to operate submerged in the liquid argon at cryogenic temperatures. The MicroBooNE experiment collected data from 2015 to 2020. Brookhaven physicists invented many new techniques to analyze the MicroBooNE data including signal processing, Wire-Cell event reconstruction, and charge-light matching. Brookhaven physicists have led several physics result analyses, including the search for low-energy excess in electron neutrinos, the measurement of the energy-dependent muon-neutrino-argon interaction cross section, and the search for an electron-volt-scale sterile neutrino with both disappearance and appearance oscillations.
The Precision Reactor Oscillation and Spectrum Experiment (PROSPECT)
The study of antineutrinos with PROSPECT allows scientists to search for a previously unobserved particle, the so-called sterile neutrino, while probing the nuclear processes inside a reactor. The experiment is installed at the High Flux Isotope Reactor (HFIR) at Oak Ridge National Laboratory and collected data in 2018. Brookhaven chemists developed and produced the detection medium that fills the detector—a unique, lithium-doped liquid scintillator. Brookhaven engineers and physicists designed the experiment’s shielding and an air-cushioned transport system that allows the 75,000-pound PROSPECT detector to be moved to different distances from the nuclear reactor to improve the experiment’s sensitivity to sterile neutrinos. The Brookhaven team, in collaboration with scientists at Temple University, developed and deployed a unique calibration technique using tiny amounts of radioactive isotopes in the liquid scintillator.
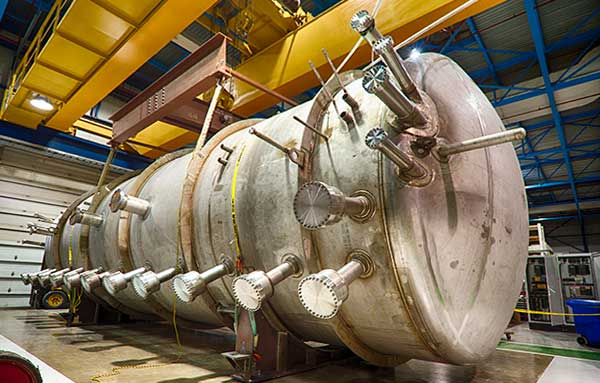
The MicroBooNE experiment cryostat. (Credit: Fermilab)
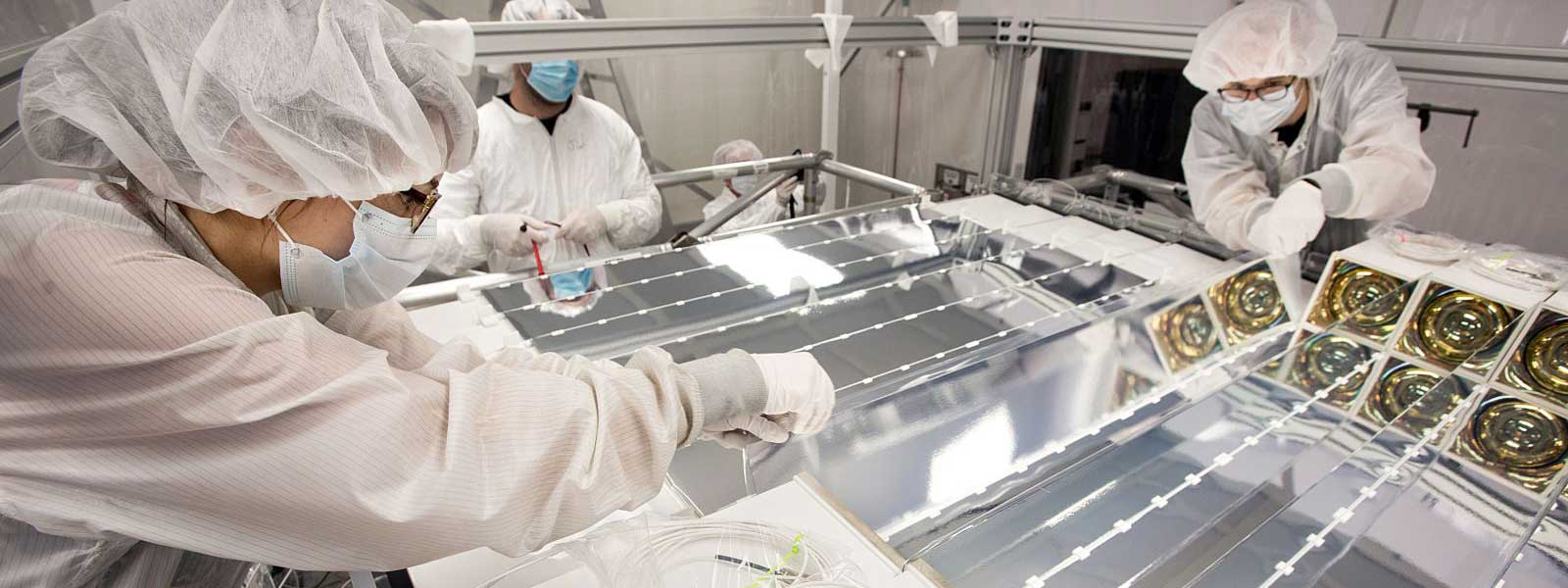
PROSPECT neutrino detector during assembly. The detector's liquid scintillator, developed at Brookhaven Lab, contained an isotope of lithium that exists in relatively low abundance in nature and is readily able to absorb neutrons produced by the antineutrino interaction.(Credit: PROSPECT collaboration / Mara Lavitt)
Imaging Cosmic And Rare Underground Signals (ICARUS) and Short-Baseline Near Detector (SBND)
The Short-Baseline Neutrino Program (SBN) at Fermilab consists of three neutrino experiments, SBND, ICARUS, and MicroBooNE, located along the Booster Neutrino Beam at different distances from the beam target. MicroBooNE completed data taking in 2020; ICARUS and SBND started operation in 2022 and 2024, respectively. The SBN program will perform sensitive searches for electron neutrino appearance and muon neutrino disappearance by comparing measurements from all three experiments. Brookhaven Lab scientists have played important roles in the LArTPC electronics readout and photon detector for ICARUS. Brookhaven physicists also have leading roles in ICARUS physics analysis, including a far-detector only measurement of muon neutrino disappearance. Brookhaven physicists and engineers played critical roles in the design, construction, operation, and calibration of SBND, including designing and building the SBND LArTPC charge-readout system, which included significant advances over MicroBooNE in the design of cold electronics.
Deep Underground Neutrino Experiment (DUNE)
In the early 2000s, Brookhaven Lab scientists conceived an experiment to produce an intense collimated broadband beam of neutrinos that would travel hundreds of miles through the Earth and strike a distant target to help unravel the mysteries of matter. Traveling over such a long distance would give the particles time to exhibit one of their strangest and most exciting quirks: quantum mechanical flavor transformations. The experiment was designed to measure that such transformations are not the same for particles and antiparticles, thus illuminating one of the most important puzzles in fundamental physics. The project was approved as the Long-Baseline Neutrino Experiment (LBNE), with the beam initiating at Fermilab and striking a very large, underground detector capable of identifying and precisely measuring neutrino events at the Sanford Underground Research Facility (SURF) in Lead, South Dakota — in the same Homestake Mine where Ray Davis did his famous experiment. Brookhaven Lab scientists have been principal collaborators in this experiment, from fundamental neutrino science to beam and detector design, prototyping, and construction. This program has now evolved into a large international enterprise known as the Deep Underground Neutrino Experiment at the Long-Baseline Neutrino Facility (DUNE at LBNF) with more than 1000 scientists, including principal collaborators from Brookhaven Lab.
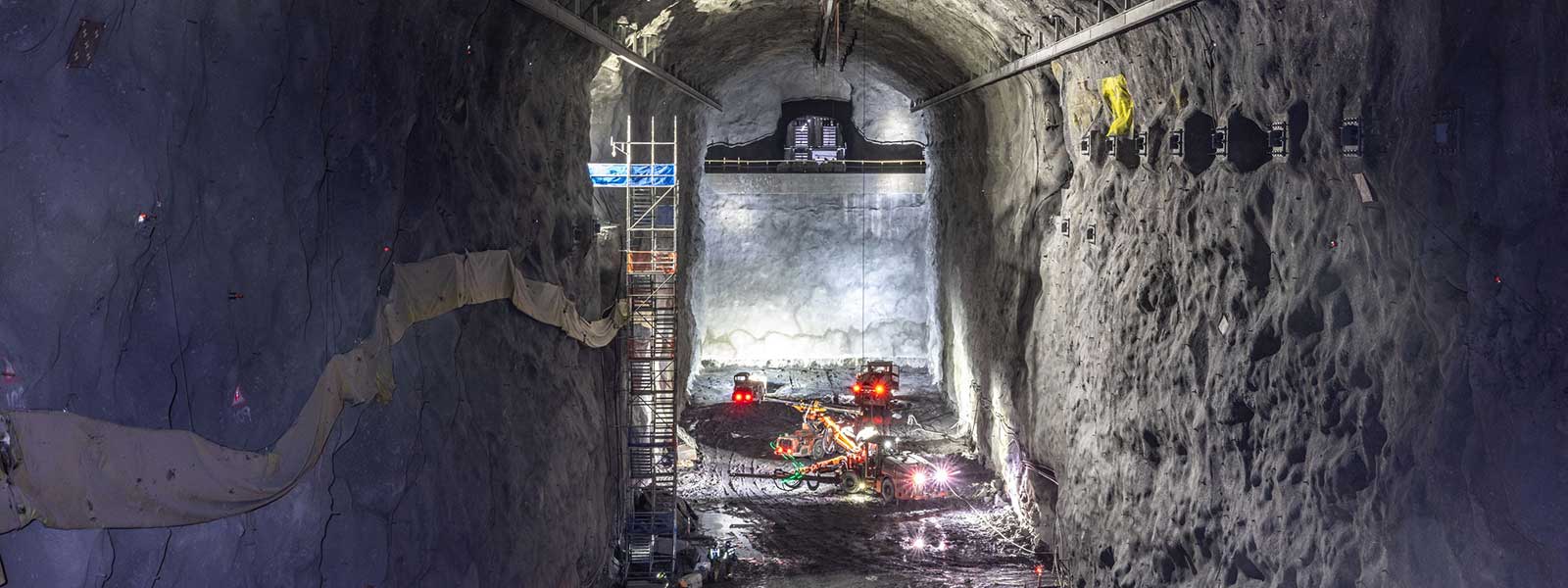
A bird's eye view of one of the large caverns in South Dakota, about the height of a seven-story building. Large particle detectors for the Deep Underground Neutrino Experiment will be placed here to study the behavior of neutrinos. The DUNE detectors are expected to be the largest underground cryogenic system in the world. (Credit): Matthew Kapust/Sanford Underground Research Facility)
Neutrino Detector Development
Brookhaven Lab has ongoing, active programs to develop neutrino detector technologies, including “cold electronics” that can operate at the cryogenic temperatures within modern neutrino detectors and the liquid argon technology that makes neutrino detection possible. The Lab also has a strong program of detector technology development based on its longstanding expertise in neutrino and nuclear chemistry.